Introduction
Liver is the largest internal organ in the body and the primary site for various metabolic and detoxification functions. Liver is vital for energy metabolism, biosynthesis, and removing toxic substances and drugs from the body [
1]. However, liver is susceptible to injury from various infectious agents, drugs, and toxins, considering its central role in metabolism and clearance. Drug-induced liver injury (DILI) is the leading cause of acute liver failure and represents a major clinical and economic burden worldwide [
2].
Acetaminophen (APAP), one of the primary drugs capable of causing DILI in therapeutic misuse or overdose scenarios, is the most commonly used analgesic and antipyretic medication in Nigeria and across Africa [
3]. The mechanism of APAP hepatotoxicity involves metabolism by cytochrome P450 enzymes to form the reactive intermediate, N-acetyl-p-benzoquinone imine (NAPQI) [
4]. While NAPQI is typically neutralized by binding to glutathione, excessive APAP doses deplete liver glutathione stores.
While N-acetylcysteine (NAC) can replenish glutathione, delayed administration beyond 8 hours after ingestion reduces its effectiveness [
5]. Furthermore, NAC may not be readily available or affordable for many Nigerian patients. Liver transplant is the only definitive treatment but is limited in Nigeria due to lack of facilities, high costs, and organ shortage [
6]. Therefore, there is an urgent need for affordable therapeutic options that can reduce APAP hepatotoxicity. Locally available medicinal plants may offer potential options.
Medicinal plants have traditionally been used worldwide to treat various ailments, and their phytochemicals can potentially be developed into effective therapies [
7]. Lantana camara L. (Verbenaceae) is a shrub that grows widely across tropical and subtropical regions as an invasive species in over 60 countries across Africa, including Nigeria [
8], Asia and Australia [
9].
L. camara has been extensively studied for its various pharmacological properties. For instance, the leaf extracts of L. camara have been found to exhibit the highest antioxidant activity due to the presence of phenolic compounds and flavonoids [
10]. The plant extract effectively reduced inflammation and edema in an animal model, demonstrating its anti-inflammatory properties [
11]. Studies have also reported significant hypoglycaemic and antihyperglycemic effects of L. camara in diabetic animal models [
12, 13]. Furthermore, the plant has shown antimicrobial properties, inhibiting the growth of various pathogenic bacteria and fungi [
9,
14, 15]. L. camara has also been studied for its antiulcerogenic [
16], wound healing [
17-
19] and antihyperglycemic activities [
20], with potential therapeutic applications in these areas.
These pharmacological activities have been attributed to its rich phytochemical constituents. Phytochemical studies have revealed that L. camara is rich in flavonoids like quercetin, kaempferol and hispidulin; phenolic compounds like caffeic acid and ferulic acid; triterpenes like ursolic acid and oleanolic acid; and alkaloids like lantamine and camarin [
9,
21]. However, there are also reports of L. camara toxicity, especially from ingestion of unripe green berries containing higher concentrations of pentacyclic triterpenoids like lantadene A and lantadene B, causing hepatotoxicity, photosensitivity, and respiratory distress in animals and humans [
22].
However, while L. camara shows potential hepatoprotective effects based on its traditional use and phytochemical composition [
23]. The present study aimed to evaluate the hepatoprotective efficacy of methanolic leaf extract of L. camara against APAP-induced hepatotoxicity in a rat model.
Materials and Methods
Plant material and extraction
The fresh leaves of L. camara were collected from wild sources at the National Veterinary Research Institute (NVRI), Vom, Nigeria (9°43’47.77’’N, 8°47›24.38››E). O. E. Agyeno, a Botanist from the Department of Plant Science Technology, University of Jos, Plateau State, performed taxonomic identification and authentication where a voucher specimen (Herbarium No. UJH19000291) was deposited. The collected leaves were cleaned and shade-dried, powdered, and subjected to methanol extraction using the maceration method described by Araya et al. [
24]. The powdered plant sample was infused in methanol for 72 hours and filtered using Whatman Filter Paper No. 1. The filtrate was dried at 50°C and stored at 4°C.
Phytochemical screening
Preliminary phytochemical screening of the extract was performed to identify major chemical constituents using standard procedures [
25]. Qualitative and quantitative evaluation of the phytochemical constituent was carried out.
Experimental drug
APAP powder (McNeil Product Ltd, SL6, UK) was suspended in 1% carboxymethyl cellulose and administered by oral gavage at 750 mg/kg body weight to induce hepatotoxicity in Wistar rats.
Experimental animals
In this study, 25 male Wistar albino rats weighing 100-150 g were procured from the National Veterinary Research Institute, Vom, Plateau State. The animals were housed under standard room temperature and 12 hours of light/dark cycle and fed with a commercial pellet diet and water ad libitum [
26].
Experimental design
The Wistar rats were divided into five groups of 5 rats each and were treated for 6 days as follows. Group I (normal control): Distilled water orally (10 mL/kg); Group II (APAP control): Distilled water for 5 days, then APAP (750 mg/kg) [
27] by oral gavage to induce hepatotoxicity on day 6 [
28]; Group III (extract 100 mg/kg): L. camara leaf extract (100 mg/kg) for 5 days, then APAP (750 mg/kg) on day 6; Group IV (extract 300 mg/kg): L. camara leaf extract (300 mg/kg) for 5 days, then APAP (750 mg/kg) on day 6; Group V (extract 500 mg/kg): L. camara leaf extract (500 mg/kg) for 5 days, then APAP (750 mg/kg) on day 6.
The vehicle, extracts and APAP were administered once daily by oral gavage in a volume of 10 mL/kg body weight. On the 6th day, 24 hours after APAP administration, the rats were anesthetized using a ketamine/xylazine cocktail, blood was collected by cardiac puncture, and liver tissues were excised after euthanasia by cervical dislocation. Serum biomarkers and histological parameters were evaluated using standard procedures.
Biochemical analysis
Serum levels of alanine transaminase (ALT) and aspartate transaminase (AST) were determined through the calorimetric method at 546 nm using Randox assay kits based on principles described by Reitman and Frankel [
29]. Alkaline phosphatase (ALP), which catalyzes the hydrolysis of the colorless organic phosphate ester substrate, p-nitrophenyl phosphate, to the yellow-colored product p-nitrophenol and phosphate [
30], was also determined using Randox assay kits, calorimetrically at 410 nm. Total and direct bilirubin levels were also determined calorimetrically at 578 nm using Randox assay kits. Direct bilirubin and diazotized sulphanilic acid were combined in an alkaline medium to generate a blue complex. By reacting with diazotized sulphanilic acid in the presence of caffeine, which releases albumin-bound bilirubin, total bilirubin is measured [
31]. Also, total protein was determined based on principles described by Weichselbaum [
32], which involves the formation of colored biuret complex and albumin level based on principles described by Doumas et al. [
33] using a Randox assay kit.
Percent hepatoprotection
The percentage of hepatoprotection against APAP-induced alterations in biochemical markers by L. camara leaf extract in a pretreatment model was assessed using Kolakota et al.’s approach [
34]. To calculate the percentage of protection, it is assumed that the APAP toxic group’s biomarker levels indicate 0% protection, and the normal control group—a group not exposed to APAP—represents 100% protection. The percentage protection was determined using the
Equation 1:

where, N refers to the mean value of negative control, T denotes the mean value of treatment groups and C equals the mean value of normal control.
Histopathological studies
The histopathological analysis of liver tissues excised from the experimental animals and fixed in 10% neutral buffered formalin was carried out as described by Choji et al. [
35].
Statistical analysis
Data were expressed as Mean±SEM and evaluated using a one-way analysis of variance (ANOVA) and Tukey post hoc test in SPSS software, version 27. P<0.05 were considered significant.
Results
Phytochemical analysis
The preliminary phytochemical screening of the methanolic leaf extract of L. camara revealed phenolics (5.03%) and alkaloids (4.30%) were found in the highest amounts, followed by saponins (6.14%), tannins (3.81%) and flavonoids (3.54%) (
Table 1).
Biochemical analysis of liver enzymes
APAP administration at 750 mg/kg significantly increased the serum activities of some hepatic enzymes, including AST, ALT and ALP, compared to normal control animals (P<0.05) (
Table 2).
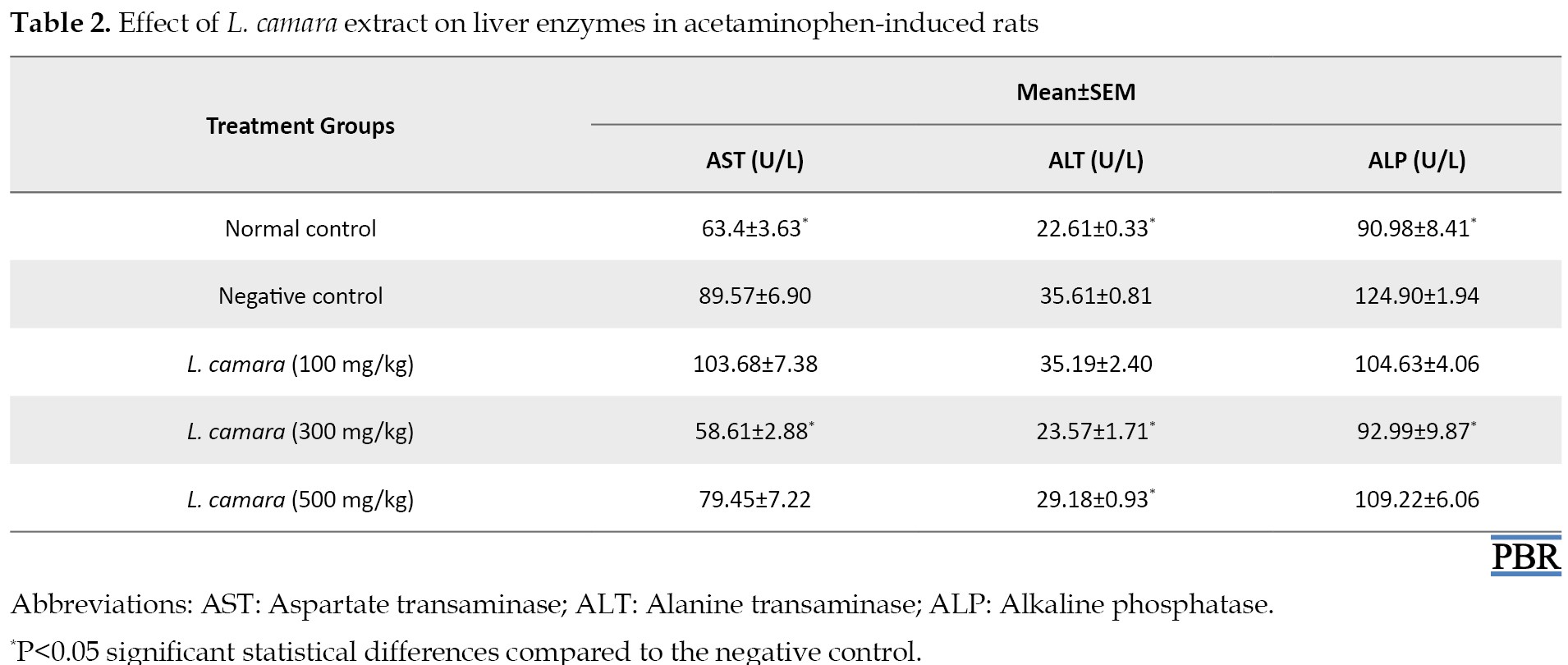
Rats treated solely with APAP showed 89.57±6.90 U/L AST level compared to 63.4±3.63 U/L in normal controls. APAP also raised ALT and ALP to 35.61±0.81 U/L and 124.90±1.94 U/L versus 22.61±0.33 U/L and 90.98±8.41 U/L in normal rats.
Pretreatment with 100 mg/kg L. camara leaf extract before the APAP challenge did not confer significant protection based on liver enzyme leakage. AST, ALT and ALP levels in this group were statistically comparable to the APAP control and were significantly higher (P<0.05) than normal rats. However, pretreatment with 300 mg/kg leaf extract markedly lowered (P<0.05) AST, ALT and ALP levels to 58.61±2.88 U/L, 23.57±1.71 U/L and 92.99±9.87 U/L, respectively. These enzyme activities were comparable to normal control levels and significantly reduced (P<0.05) relative to the APAP group. The 500 mg/kg extract dose also showed partial restoration by lowering ALT to 29.18±0.93 U/L versus 35.61±0.81 U/L in the APAP control (P<0.05). However, AST and ALP levels were not significantly different from the injury model at this dose.
As shown in
Figure 1, a 300 mg/kg dose of L.
camara leaf extract exhibited maximal protection against APAP-induced leakage of liver enzymes AST, ALT and ALP, conferring 100%, 92% and 94% protection, respectively. Also, the 100 mg/kg extract dose did not result in significant enzymatic protection, while the 500 mg/kg dose provided intermediate protection of 39% for AST and around 50% for ALT and ALP.
Biochemical analysis of total protein, albumin, and bilirubin
The administration of APAP caused a significant reduction in serum total protein and albumin levels compared to normal control animals (
Table 3).

The APAP group had a total protein of 57.77±0.73 g/L versus 63.00±1.41 g/L in controls. Serum albumin was decreased to 25.21±1.08 g/L relative to 22.92±1.60 g/L in normal rats. Pretreatment with 100 mg/kg L. camara leaf extract did not restore total protein or albumin, with levels comparable to the APAP group (P<0.05). However, the 300 mg/kg extract dose significantly increased total protein and albumin close to normal control values (P<0.05). The 500 mg/kg dose, however, does not attenuate the APAP-induced hypoalbuminemia and hypoproteinaemia.
Total and direct bilirubin were significantly higher in the APAP group compared to normal controls (P<0.05) (
Table 3). Pretreatment with 100 mg/kg extract failed to prevent hyperbilirubinemia, with total and direct bilirubin comparable to the APAP group. However, the 300 and 500 mg/kg extract doses significantly attenuated the APAP-induced increase in direct bilirubin (P<0.05). Total bilirubin was also lowered by these doses, although not to the extent of direct bilirubin. The results indicate a dose-dependent improvement in conjugation and excretory capacity of the liver conferred by L. camara leaf extract against APAP toxicity.
Analysis of protection against APAP-mediated declines in serum proteins and bilirubin is shown in
Figure 2.
The 100 mg/kg extract dose did not significantly protect serum biochemical parameters. Also, 300 mg/kg extract dose demonstrated high protection of 95%, 100%, and 83% for serum albumin, total protein, and direct bilirubin, respectively. The highest dose of 500 mg/kg provided complete reversal of increased total bilirubin and partial restoration of direct bilirubin and albumin.
Histopathological analysis
Figures 3 and
4 show the histological changes at ×100 and ×400 magnification. Microscopic observation of normal control liver sections showed the characteristic hexagonal hepatocytes with granulated cytoplasm and centrally located nuclei (
Figures 3A and
4A).
In contrast, the APAP-treated group exhibited extensive hepatocellular necrosis with derangement of the radial arrangement of the hepatocytes along with sinusoidal dilatation. Cellular demarcations appear as strands, while mild hemorrhage is demonstrated by red blood cells within the tissue (
Figures 4A and
4B). Cytoplasmic vacuolization, nuclear pyknosis, and disrupted cell membranes were visible, indicating severe hepatic injury. Pretreatment with 100 mg/kg L. camara leaf extract could not prevent APAP-induced necrosis, with microscopic features similar to the APAP control group (
Figures 3C and
4C). Extensive hepatocellular degeneration, apoptotic nuclei, and necrotic debris were observed. The 300 mg/kg extract dose conferred moderate protection of the lobular architecture and hepatocytes (
Figures 3D and
4D). Mild sinusoidal dilation and occasional necrosis were visible, but overall cytoarchitecture was preserved. Nuclei were intact and radially arranged around the central vein. Sections of the 500 mg/kg extract pretreatment group showed near-normal lobular patterns with minimal disruption or necrosis (
Figures 3E and
4E). Mild lymphocytic infiltration was observed, indicating some cellular inflammatory response.
Discussion
This study evaluated the hepatoprotective potential of L. camara, a medicinal plant used traditionally against liver ailments [
36]. Phytochemical analysis revealed the presence of various bioactive compounds like phenolics, flavonoids, alkaloids, saponins and tannins in L. camara leaf extract. Plant phenolics exhibit a wide range of biological properties, including antioxidant, anti-inflammatory, antimicrobial, immunomodulatory, and tissue-protective effects [
37]. The phenolic compounds in L. camara leaves can scavenge free radicals, chelate redox-active metals, and attenuate lipid peroxidation and protein carbonylation induced by toxins like APAP [
38]. This property may significantly contribute to its observed hepatoprotective activity. Likewise, flavonoids can prevent hepatocellular damage through antioxidant mechanisms as well as via modulating cytochrome P450 enzymes involved in xenobiotic metabolism and bioactivation [
39, 40]. Saponins have also shown protective effects against DILI by preserving membrane integrity and enhancing glutathione redox cycling [
41]. Alkaloids, though toxic at high doses, may stimulate tissue repair processes at lower concentrations by regulating growth factors, cytokines, and extracellular matrix remodeling [
42]. Similarly, tannins can promote hepatocyte regeneration and accelerate normalization of liver function [
43].
APAP overdose caused extensive liver injury as evidenced by marked elevation of serum AST, ALT and ALP levels, comparable to other studies using similar APAP doses in rats [
44, 45]. These membrane-bound enzymes are released into circulation following hepatic cellular damage, making them clinically relevant markers of hepatocellular injury [
46]. However, the dramatic AST increases in this study are likely from the liver due to simultaneous ALT increases. The profound rise in their serum activities indicates considerable membrane fragility and leakage of these cytosolic enzymes into circulation. Elevated ALP levels correlate with cholestatic liver damage as this enzyme is abundantly present on the canalicular membrane of hepatocytes and biliary epithelial cells [
47]. Pretreatment with 300 mg/kg L. camara leaf extract remarkably restored AST, ALT and ALP to normal control levels, indicating superior hepatoprotective efficacy compared to extracts of Aerva javanica [
48] or Acacia catechu [
49], which offered only partial protection at higher doses, signifying profound stabilization of hepatocellular membrane architecture and structural integrity. This membrane-protective effect can be attributed to the antioxidant phytochemicals like phenolics and flavonoids present in the extract [
50]. This condition attenuates membrane disruption and the resultant leakage of liver enzymes into circulation. The 300 mg/kg dose likely represents an optimal balance and synergy between the different hepatoprotective phytochemicals in L. camara leaves. In contrast, at 500 mg/kg, the inhibitory effects of less active components may predominate, resulting in reduced efficacy [
11].
The significant decline in serum total protein and albumin signifies reduced hepatic protein synthesis due to APAP-induced liver injury [
51]. Albumin comprises over half of the total measured serum proteins and is exclusively synthesized in the liver. Therefore, hypoalbuminemia is a clinical marker of diminished liver function and impaired hepatocellular protein production capacity [
51]. Pretreatment with 300 mg/kg L. camara leaf extract optimally restored these serum protein and bilirubin parameters close to normal control levels. The phytochemicals may stimulate regeneration mechanisms, preserve functional hepatocytes, and enhance bile flow to normalize serum biomarkers [
9].
Microscopic observation of liver sections provided visual evidence of the biochemical and functional alterations induced by APAP overdose. Extensive centrilobular necrosis, sinusoidal dilation, cytoplasmic vacuolization, and nuclear pyknosis seen in the APAP group correlate with the severe membrane and mitochondrial damage caused by oxidative stress and protein binding of NAPQI [
1]. Loss of cellular architecture and accumulation of necrotic debris signify profound structural disruption of the hepatocyte cytoskeleton [
4]. This effect complements the marked leakage of liver enzymes into circulation. The 100 mg/kg L. camara leaf extract dose could not prevent this damage. However, pretreatment with 300 and 500 mg/kg L. camara leaf extract markedly reduced this morphological degradation, maintaining near-normal liver tissue and cell structure. The extracts likely preserve membrane integrity, stimulate tissue repair, and enhance removal of necrotic cells to facilitate regeneration [
52].
This research demonstrates the hepatoprotective potential of the medicinal shrub L. camara. The study used a rat model to show that pretreatment reduced this morphological degradation, maintaining near-normal liver tissue and cell structure. The extracts likely preserve membrane integrity, stimulate tissue repair and enhance removal of necrotic cells to facilitate regeneration [
52].
This study evaluated hepatoprotection in a pretreatment model, which differs from the more common post-treatment approach used for many natural products [
24,
48]. The pretreatment regimen assesses the extract’s ability to induce cellular defense mechanisms and prepare the liver to withstand the subsequent toxic insult [
53]. This approach is clinically relevant for high-risk patients prone to DILI.
Conclusion
This research demonstrates the hepatoprotective potential of the medicinal shrub L. camara. The study used a rat model to show that pretreatment with L. camara leaf extract can mitigate liver damage caused by an overdose of APAP. The extract was particularly effective at 300 mg/kg, normalizing liver enzyme activities and maintaining liver functional biomarkers. It also maintained near-normal liver tissue architecture with minimal necrosis by likely preserving hepatocellular membrane integrity and stimulating regeneration.
Ethical Considerations
Compliance with ethical guidelines
Animals were handled according to the Research Animal Ethics Committee of Modibbo Adama University (Code: UAEC/YMAU/YL/0753) as per prescribed guidelines by the National Health Research Ethics Committee of Nigeria on Care and Use of Laboratory Animals.
Funding
This research did not receive any grant from funding agencies in the public, commercial, or non-profit sectors.
Authors' contributions
Experimentation: Hyelda Stephen; Study design, literature review, data interpretation, analysis and writing: All authors.
Conflict of interest
The authors declared no conflict of interest.
Acknowledgments
The authors thank the Biochemistry Division of the National Veterinary Research Institute (NVRI), Vom, Plateau State, for providing the facilities and support to carry out this study and acknowledge the technical support provided by Choji Tobias Peter Pwajok of Central Diagnostics, NVRI, for his expertise and assistance with the histopathological analysis, as well as Daniel Shailong in the animal experiments and biochemical assays.
References
- García-Román R, Francés R. Acetaminophen-induced liver damage in hepatic steatosis. Clin Pharmacol Ther. 2020; 107(5):1068-81. [DOI:10.1002/cpt.1701] [PMID]
- Francis P, Navarro VJ. Drug induced hepatotoxicity. Treasure Island: StatPearls; 2020. [Link]
- Adedeji WA, Dairo MD, Nguku PM, Oyemakinde A, Fehintola FA. Pattern and predictors of medication use among adults in southwestern Nigeria: A community-based cross-sectional study. Pharmacol Res Perspect. 2023; 11(1):e01017. [DOI:10.1002/prp2.1017] [PMID] [PMCID]
- Ramachandran A, Jaeschke H. Acetaminophen hepatotoxicity. Semin Liver Dis. 2019; 39(2):221-34. [DOI:10.1055/s-0039-1679919] [PMID] [PMCID]
- Akakpo JY, Ramachandran A, Curry SC, Rumack BH, Jaeschke H. Comparing N-acetylcysteine and 4-methylpyrazole as antidotes for acetaminophen overdose. Arch Toxicol. 2022; 96(2):453-65. [DOI:10.1007/s00204-021-03211-z] [PMID] [PMCID]
- Offor SJ, Amadi CN, Chijioke-Nwauche I, Manautou JE, Orisakwe OE. Potential deleterious effects of paracetamol dose regime used in Nigeria versus that of the United States of America. Toxicol Rep. 2022; 9:1035-44. [DOI:10.1016/j.toxrep.2022.04.025] [PMID] [PMCID]
- Nguyen-Vo TH, Nguyen L, Do N, Nguyen TN, Trinh K, Cao H, et al. Plant metabolite databases: from herbal medicines to modern drug discovery. J Chem Inf Model. 2020; 60(3):1101-10. [DOI:10.1021/acs.jcim.9b00826] [PMID]
- Agaldo JA. Ecology of lantana camara: An invasive plant species in amurum forest reserve, Jos Plateau, Central Nigeria. Afr J Ecol. 2020; 58:291-8. [DOI:10.1111/aje.12698]
- Battase D, Attarde D. Phytochemical and medicinal study of Lantana camara Linn.(Verbenaceae)-A review. Asian J Pharm Clin Res. 2021; 14(9):20-7. [DOI:10.22159/ajpcr.2021.v14i9.42444]
- Bhakta D, Ganjewala D. Effect of leaf positions on total phenolics, flavonoids and proanthocyanidins content and antioxidant activities in Lantana camara (L). J Sci Res. 2009; 1(2):363-9. [DOI:10.3329/jsr.v1i2.1873]
- Sore MA, Mwonjoria JK, Juma KK, Piero NM, Mwaniki NE. Evaluation of analgesic, anti-inflammatory and toxic effects of Lantana camara L. Int J Phytopharmacol. 2017; 8(3):89-97. [Link]
- Balti T, Charradi K, Mahmoudi M, Oueslati N, Limam F, Aouani E. Paradoxical anti-diabetic effect of lantana camara leaf extract and pancreatic oxidative stress relieved by grape seed and skin extract. Pharm Chem J. 2022; 55:1219-28. [DOI:10.1007/s11094-022-02561-x]
- Venkatachalam T, Kumar VK, Selvi PK, Maske AO, Anbarasan V, Kumar PS. Antidiabetic activity of Lantana camara Linn fruits in normal and streptozotocin-induced diabetic rats. J Pharm Res. 2011; 4(5):1550-2. [Link]
- Ajayi OO, Aborode AT, Subuloye OI, Ajagbe AO, Sillanpaa M, Ogemdi IK, et al. Antibacterial Activity Studies of Essential Oils from Red Sage (Lantana Camara). 2022; [Unpublished]. [DOI:10.21203/rs.3.rs-1299145/v1]
- Ganjewala D, Sam S, Khan K. Biochemical compositions and antibacterial activities of Lantana camara plants with yellow, lavender, red and white flowers. Eurasia J Biosci. 2013; 3:69-77. [Link]
- Thamotharan G, Sekar G, Ganesh T, Sen S, Chakraborty R, Kumar S. Antiulcerogenic effects of Lantana camara Linn. leaves On in vivo test models in rats. Asian J Pharm Clin Res. 2010; 3(4):57-60. [Link]
- Mahmood Ameen Abdulla MA, Pouya Hassandarvish PH, Hapipah Mohd Ali HM, Suzita Mohd Noor SM, Fouad Hussain Mahmoud FH, Nor Syuhada AB, et al. Acceleration of wound healing potential by Lantana camara leaf extract in experimental rats. Res J Med Sci. 2009; 3(2):75-9. [Link]
- Nayak BS, Raju SS, Ramsubhag A. Investigation of wound healing activity of Lantana camara L. in Sprague dawley rats using a burn wound model. Int J Appl Res Nat Pro. 2008; 1(1):15-9. [Link]
- Nayak BS, Raju SS, Eversley M, Ramsubhag A. Evaluation of wound healing activity of Lantana camara L. - a preclinical study. Phytother Res. 2009; 23(2):241-5. [DOI:10.1002/ptr.2599] [PMID]
- Ganesh T, Sen S, Thilagam E, Thamotharan G, Loganathan T, Chakraborty R. Pharmacognostic and antihyperglycemic evaluation of Lantana camara (L.) var. aculeate leaves in alloxan-induced hyperglycemic rats. Int J Res Pharm. 2010; 1(3):247-52. [Link]
- Ono M, Hashimoto A, Miyajima M, Sakata A, Furusawa C, Shimode M, et al. Two new triterpenoids from the leaves and stems of Lantana camara. Nat Prod Res. 2021; 35(21):3757-65. [DOI:10.1080/14786419.2020.1736063] [PMID]
- Kumar R, Katiyar R, Kumar S, Kumar T, Singh V. Lantana camara: An alien weed, its impact on animal health and strategies to control. J Exp Biol. 2016; 4(3S):321-36. [DOI:10.18006/2016.4(3S).321.337]
- Grace-Lynn C, Darah I, Chen Y, Latha LY, Jothy SL, Sasidharan S. In vitro antioxidant activity potential of lantadene A, a pentacyclic triterpenoid of Lantana plants. Molecules. 2012; 17(9):11185-98. [DOI:10.3390/molecules170911185] [PMID] [PMCID]
- Araya EM, Adamu BA, Periasamy G, Sintayehu B, Gebrelibanos Hiben M. In vivo hepatoprotective and In vitro radical scavenging activities of Cucumis ficifolius A. rich root extract. J Ethnopharmacol. 2019; 242:112031. [DOI:10.1016/j.jep.2019.112031] [PMID]
- Evans WC. Trease and evans pharmacognosy. Amsterdam: Elsevier; 2009. [Link]
- Federal Ministry of Health. National code for health research ethics. Abuja: Federal Ministry of Health; 2014. [Link]
- Hakkim FL, Bakshi HA, Khan S, Nasef M, Farzand R, Sam S, et al. Frankincense essential oil suppresses melanoma cancer through down regulation of Bcl-2/Bax cascade signaling and ameliorates heptotoxicity via phase I and II drug metabolizing enzymes. Oncotarget. 2019; 10(37):3472-90. [DOI:10.18632/oncotarget.26930] [PMID] [PMCID]
- Mansourian M, Mirzaei A, Azarmehr N, Vakilpour H, Kokhdan EP, Doustimotlagh AH. Hepatoprotective and antioxidant activity of hydroalcoholic extract of Stachys pilifera. Benth on acetaminophen-induced liver toxicity in male rats. Heliyon. 2019; 5(12):e03029. [DOI:10.1016/j.heliyon.2019.e03029] [PMID] [PMCID]
- Reitman S, Frankel S. A colorimetric method for the determination of serum glutamic oxalacetic and glutamic pyruvic transaminases. Am J Clin Pathol. 1957; 28(1):56-63. [DOI:10.1093/ajcp/28.1.56] [PMID]
- Roy AV. Rapid method for determining alkaline phosphatase activity in serum with thymolphthalein monophosphate. Clin Chem. 1970; 16(5):431-6. [DOI:10.1093/clinchem/16.5.431] [PMID]
- Jendrassik L, Grof P. Quantitative determination of total and direct bilirubin in serum and plasma. Biochem Z. 1938; 297:81-9.
- Weichselbaum CT. An accurate and rapid method for the determination of proteins in small amounts of blood serum and plasma. Am J Clin Pathol. 1946; 16(3):40-9. [DOI:10.1093/ajcp/16.3_ts.40]
- Doumas BT, Watson WA, Biggs HG. Albumin standards and the measurement of serum albumin with bromcresol green. Clin Chim Acta. 1971; 31(1):87-96. [DOI:10.1016/0009-8981(71)90365-2] [PMID]
- Kolakota R, Kumar RS, Patnaik SK. In vitro antioxidant activity and hepatoprotective potential of ceropegia spiralis against paracetamol induced liver injury. J Appl Pharmaceutical Sci. 2017; 7(9):199-206. [DOI:10.7324/JAPS.2017.70927]
- Choji TPP, Ngokere AA, Ogenyi SI, Kumbish PR. Histoarchitectural evaluation of conventional versus two rapid microwave processing techniques. Br Biotechnol J. 2015; 8(3):1-19. [DOI:10.9734/BBJ/2015/18948]
- Katiyar S, Patidar D, Gupta S, Singh R, Singh P. Some Indian traditional medicinal plants with antioxidant activity: A review. Int J Innovat Res Sci Eng Technol. 2013; 2(12):7303-14. [Link]
- Chiorcea-Paquim AM, Enache TA, De Souza Gil E, Oliveira-Brett AM. Natural phenolic antioxidants electrochemistry: Towards a new food science methodology. Compr Rev Food Sci Food Saf. 2020; 19(4):1680-726. [DOI:10.1111/1541-4337.12566] [PMID]
- Sotler R, Poljšak B, Dahmane R, Jukić T, Pavan Jukić D, Rotim C, et al. Prooxidant activities of antioxidants and their impact on health. Acta Clin Croat. 2019; 58(4):726-36. [DOI:10.20471/acc.2019.58.04.20] [PMID] [PMCID]
- Chen CH. Xenobiotic metabolic enzymes: Bioactivation and antioxidant defense. Berlin: Springer; 2020. [DOI:10.1007/978-3-030-41679-9]
- Ekpo DE, Joshua PE, Odiba AS, Nwodo OFC. Flavonoid-rich fraction of Lasianthera africana leaves alleviates hepatotoxicity induced by carbon tetrachloride in Wistar rats. Drug Chem Toxicol. 2022; 45(5):1934-50. [DOI:10.1080/01480545.2021.1892957] [PMID]
- Adetuyi BO, Adebisi OA, Adetuyi OA, Ogunlana OO, Toloyai PE, Egbuna C, et al. Ficus exasperata attenuates acetaminophen-induced hepatic damage via NF-κB signaling mechanism in experimental rat model. Biomed Res Int. 2022; 2022:6032511. [DOI:10.1155/2022/6032511] [PMID] [PMCID]
- Dudala SS, Venkateswarulu TC, Kancharla SC, Kodali VP, Babu DJ. A review on importance of bioactive compounds of medicinal plants in treating idiopathic pulmonary fibrosis (special emphasis on isoquinoline alkaloids). Future J Pharm Sci. 2021; 7:1-20. [DOI:10.1186/s43094-021-00304-5]
- Chen P-Y, Liao Y-H, Huang W-T, Lin Y-C, Hou Y-T. Effects of tannic acid on liver function in a small hepatocyte-based detachable microfluidic platform. Biochem Eng J. 2023; 190:108757. [DOI:10.1016/j.bej.2022.108757]
- Devkar ST, Kandhare AD, Zanwar AA, Jagtap SD, Katyare SS, Bodhankar SL, et al. Hepatoprotective effect of withanolide-rich fraction in acetaminophen-intoxicated rat: Decisive role of TNF-α, IL-1β, COX-II and iNOS. Pharm Biol. 2016; 54(11):2394-403. [DOI:10.3109/13880209.2016.1157193] [PMID]
- Omidi A, Riahinia N, Montazer Torbati MB, Behdani MA. Hepatoprotective effect of Crocus sativus (saffron) petals extract against acetaminophen toxicity in male Wistar rats. Avicenna J Phytomed. 2014; 4(5):330-6. [PMID] [PMCID]
- Zhang LX, Lv Y, Xu AM, Wang HZ. The prognostic significance of serum gamma-glutamyltransferase levels and AST/ALT in primary hepatic carcinoma. BMC Cancer. 2019; 19(1):841. [DOI:10.1186/s12885-019-6011-8] [PMID] [PMCID]
- Zollner G, Fickert P, Zenz R, Fuchsbichler A, Stumptner C, Kenner L, et al. Hepatobiliary transporter expression in percutaneous liver biopsies of patients with cholestatic liver diseases. Hepatology. 2001; 33(3):633-46. [DOI:10.1053/jhep.2001.22646] [PMID]
- Arbab AH, Parvez MK, Al-Dosari MS, Al-Rehaily AJ, Ibrahim KE, Alam P, et al. Therapeutic efficacy of ethanolic extract of Aerva javanica aerial parts in the amelioration of CCl4-induced hepatotoxicity and oxidative damage in rats. Food Nutr Res. 2016; 60:30864. [DOI:10.3402/fnr.v60.30864] [PMID] [PMCID]
- Lakshmi T, Sri Renukadevi B, Senthilkumar S, Haribalan P, Parameshwari R, Vijayaraghavan R, et al. Seed and bark extracts of Acacia catechu protects liver from acetaminophen induced hepatotoxicity by modulating oxidative stress, antioxidant enzymes and liver function enzymes in Wistar rat model. Biomed Pharmacother. 2018; 108:838-844. [DOI:10.1016/j.biopha.2018.08.077] [PMID]
- El-Shafey MM, Abd-Allah GM, Mohamadin AM, Harisa GI, Mariee AD. Quercetin protects against acetaminophen-induced hepatorenal toxicity by reducing reactive oxygen and nitrogen species. Pathophysiology. 2015; 22(1):49-55. [DOI:10.1016/j.pathophys.2014.12.002] [PMID]
- Soeters PB, Wolfe RR, Shenkin A. Hypoalbuminemia: Pathogenesis and Clinical Significance. JPEN J Parenter Enteral Nutr. 2019; 43(2):181-93. [DOI:10.1002/jpen.1451] [PMID] [PMCID]
- Telles-Correia D, Barbosa A, Cortez-Pinto H, Campos C, Rocha NB, Machado S. Psychotropic drugs and liver disease: A critical review of pharmacokinetics and liver toxicity. World J Gastrointest Pharmacol Ther. 2017; 8(1):26-38. [DOI:10.4292/wjgpt.v8.i1.26] [PMID] [PMCID]
- Emad AM, Ali SF, Abdel-Rahman EA, Meselhy MR, Farag MA, Ali SS, et al. Anti-inflammatory and antioxidant effects of Apium graveolens L. extracts mitigate against fatal acetaminophen-induced acute liver toxicity. J Food Biochem. 2020; e13399. [DOI:10.1111/jfbc.13399] [PMID]