Introduction
Sodium metabisulfite (NaMBS) is employed in the food, beverage, cosmetic, medical, rubber, and photographic sectors, functioning as a preservative, bleaching, and antioxidative agent [
1]. It holds significant importance as a preservation method for various items, such as wine, beer, grapefruit juice, dried fruits, and seafood. It has widespread usage in the production of processed foods [
2]. In certain circumstances, sodium metabisulfite exhibits properties that counteract peroxide effects, creating harmful oxidizing agents [
3].
NaMBS demonstrates diverse impacts. For instance, higher doses and prolonged exposure to NaMBS are linked to reduced root growth, decreased mitotic index, and increased chromosomal abnormalities [
4]. NaMBS can potentially induce significant detrimental effects on healthy human cells through various mechanisms, including initiating apoptosis [
5]. Consumption of sub-chronic amounts of sodium metabisulfite leads to changes in immune response and shifts in biochemical, hematological, and physiological parameters in Wistar rats [
6].
Malondialdehyde (MDA) concentrations exhibited a notable rise in rats subjected to NaMBS treatment [
7]. Similarly, NaMBS triggers lipid peroxidation and prompts apoptosis in rats [
8]. Regarding its impact on potassium currents in isolated CA1 pyramidal neurons of the rat hippocampus, NaMBS displayed a concentration-dependent elevation in transient outward and delayed rectifier potassium currents [
9]. The influence of NaMBS on apoptosis within the experimental model of Parkinson disease suggests that sulfite does not exacerbate the activity of caspase-3 in the context of a 6-hydroxydopamine-induced Parkinson disease model [
10]. According to a study exploring the effects of NaMBS on locomotor activity in the Parkinson disease experimental model, sulphite does not aggravate the activity of cyclooxygenase or the levels of prostaglandin E2 or nuclear factor kappa B in a 6-hydroxydopamine-induced Parkinson disease model [
11]. Sodium metabisulfite is associated with neuronal toxicity due to its ability to heighten neuronal excitability [
12].
The hippocampus, the prefrontal cortex, and interconnected networks of neurons play roles in various aspects of cognitive and memory functions. The hippocampus-prefrontal pathway innervates the prelimbic/medial orbital areas of the prefrontal cortex in the rat [
13]. Previous studies have reported the effects of NaMBS on specific regions of a rat’s brain. However, these effects are not gender specific. For example, NaMBS was said to have induced structural changes in the medial prefrontal cortex [
1]. Meng and Nie [
9] reported that NaMBS increased potassium and sodium currents in rat hippocampal neurons, potentially decreasing their excitability. Furthermore, Lai et al. [
14] reported that NaMBS stimulated sodium currents and increased cellular excitability in excitable cells. Cumulatively, prior research has demonstrated that sub-chronic exposure to NaMBS can have intricate and potentially detrimental impacts on animal brain structure and function. However, a deficiency or scarcity of data exists regarding certain brain regions and cognitive effects. Additionally, there is a limited understanding of the gender-specific effects of NaMBS on specific brain regions. Recognizing the observed gaps in knowledge, this study explores the potential effects of sub-chronic NaMBS exposure on the hippocampus and prefrontal cortex in female Wistar rats.
Materials and Methods
Study animals
A total of 24 adolescent female Wistar rats (mean weight of 206.7 g) were acquired from Temilola Animal Husbandry in Ogbomoso, Oyo State, Nigeria. These rats were accommodated within the Faculty of Basic Medical Sciences animal facility at Adeleke University in Ede. The rats were housed in conventional plastic rat cages equipped with iron lids. A 7-day acclimatization period was observed before categorization and the initiation of substance administration. The rats were maintained under consistent, equal dark-light cycles throughout this acclimatization period and until the experiment’s conclusion. Additionally, the rats were provided unrestricted access to food and water, allowing them to consume as desired.
Experimental design
Following the acclimatization phase, the rats were allocated randomly into four sets, each containing six rats. Over 28 consecutive days, the treatments were administered through oral gavage in the following manner: Group 1 (control) was given 0.5 mL of normal saline; group 2 received NaMBS at a dosage of 100 mg/kg of body weight; group 3 was administered NaMBS at a dosage of 300 mg/kg of body weight; and group 4 received NaMBS at a dosage of 500 mg/kg of body weight.
Behavioural tests
The behavioral assessments took place from 8:00 AM to 12:00 PM, beginning a day after the conclusion of the treatment session.
Y-maze test
The Y-maze test evaluates rodents’ spatial working and reference memory, capitalizing on their inherent exploratory behavior. The testing procedure was based on a modified approach outlined by Kraeuter et al. [
15]. This method employed a maze with three arms designated as A, B, and C. Throughout the test, the rats were observed for 5 min while they freely navigated the maze. Working memory was evaluated by counting the instances of alternations, which refer to successive entries into all three arms without revisiting the same arm consecutively. Following the test, video analysis was conducted to tally the number of arm entries and alternations, enabling the computation of alternation frequency. A heightened alternation frequency indicated enhanced memory performance, signifying that the rat retained recollection of previously explored arms.
Tissue collection
Following the conclusion of the behavioral assessment, the rats designated for histopathological analysis were anesthetized through intraperitoneal injection of ketamine (50 mg/mL, Pakson Pharma, PVT Ltd., India). Subsequently, perfusion fixation was carried out using 4% paraformaldehyde (PFA), aligning with the protocol outlined by Gage et al. [
16]. The rats were decapitated, and the hippocampi and prefrontal cortex were meticulously removed from both hemispheres. These excised brain regions were subjected to a post-fixation process in 4% PFA for 18 h.
For the neurochemical analyses, the rats were humanely euthanized through cervical dislocation, followed by decapitation. This method of euthanasia was selected to prevent any potential impact of anesthetic agents on the specific neurochemicals under investigation. The hippocampi and prefrontal cortices from both hemispheres were carefully removed and homogenized in a 0.1 M phosphate buffer with a pH of 8.0, employing a Teflon Potter–Elvehjem homogenizer. The resultant homogenates underwent centrifugation at 4000 rpm for 10 min. Subsequently, the supernatants were separated and transferred into test tubes to assess MDA and acetylcholinesterase (AchE) levels.
Malondialdehyde assay
In this procedure, MDA H3 PO4 (1%, 3 mL) and aqueous thiobarbituric acid solution (0.6%, 3 mL) were combined with a 10% homogenate (0.5 mL). The mixture underwent shaking and was heated in a boiling-water bath for 45 min. After cooling, n-butanol (4 mL) was added, and the mixture was shaken again. Centrifugation at 1200 g for 15 min separated the n-butanol layer, and its optical density was measured using a spectrophotometer, utilizing absorption wavelengths of 520 nm. The concentration of MDA was calculated and expressed as µmol MDA per gram of wet tissue.
Acetylcholinesterase assay
The activity of AchE was assessed by employing the colorimetric method outlined in the work of Ellman et al. [
17]. The absorbance measurement was taken at a wavelength of 412 nm, and the absorbance was quantified in units of nmol/L.
Histopathological study
Following an 18-h post-fixation in 4% PFA, the tissue specimens underwent processing and were embedded in paraffin blocks. These tissue blocks were then cut into sections and subjected to hematoxylin and eosin (H&E) staining, following the protocols outlined by Bancroft and Gamble [
18], to perform standard histological analysis of the hippocampus and prefrontal cortex. The stained tissue sections were observed using light microscopy, utilizing the Olympus model (XSZ-107 BN [New Jersey, USA] and Amscope, MD500) [
19].
Immuno-histochemistry assay
Tissue sections underwent deparaffinization and antigen retrieval, followed by treatment with phosphate-buffered saline and hydrogen peroxide to block endogenous peroxidase activity. Background staining was suppressed with normal goat serum, and then sections were incubated with polyclonal rabbit anti-glial fibrillary acidic protein (GFAP) serum (diluted 1:500) for 24 h at 4°C. Biotinylated mouse anti-rabbit solution and the Avidin-Biotin complex were applied successively, followed by visualization of the immunocomplex using 3,3’-diaminobenzidine. Haematoxylin counterstaining was performed, and the sections were observed under light microscopy at a scale bar of 180 µ.
Morphometric analyses
Morphometric analyses were performed using the ImageJ software, version 1.52r, on sections stained with H&E and GFAP immunostaining. Five sections from six distinct visual fields were assessed in each tissue block, spanning magnifications ranging from 180 µm. For H&E-stained sections, the count of pyramidal cells (PCs) within the CA1 region and layer II/III of the prefrontal cortex was conducted for all groups. Moreover, GFAP-immunoreactive astrocytes were quantified in the CA1 and dentate gyrus regions. The criteria for counting GFAP-immuno-positive cells were based on clearly identifiable cell bodies with extended processes, following the methodology outlined by a previous study [
20].
Statistical analyses
Data analysis was conducted using GraphPad Prism software, version 7. The statistical comparisons involved utilizing a one-way analysis of variance in combination with the Tukey multiple comparison test. All outcomes were presented as Mean±SD. In addition, statistical significance was determined at a threshold of P<0.05.
Results
Changes in learning and memory in the study groups (number of complete alternations)
The number of complete alternation from the Y-maze test (
Table 1) shows no statistically significant difference (P>0.05) between the treated groups (100 mg/kg, 300 mg/kg, 50 mg/kg) and the control group (2.3±0.4; 2.3±0.47; 1.7±0.4 vs 2.7±0.47).
Variations in malondialdehyde and acetylcholinesterase levels
MDA concentration (
Table 2) demonstrates no notable statistical distinction (P>0.05) between the groups subjected to treatment and the control group (0.59±0.13; 0.64±0.26; 0.68±0.13 vs. 0.48±0.04).
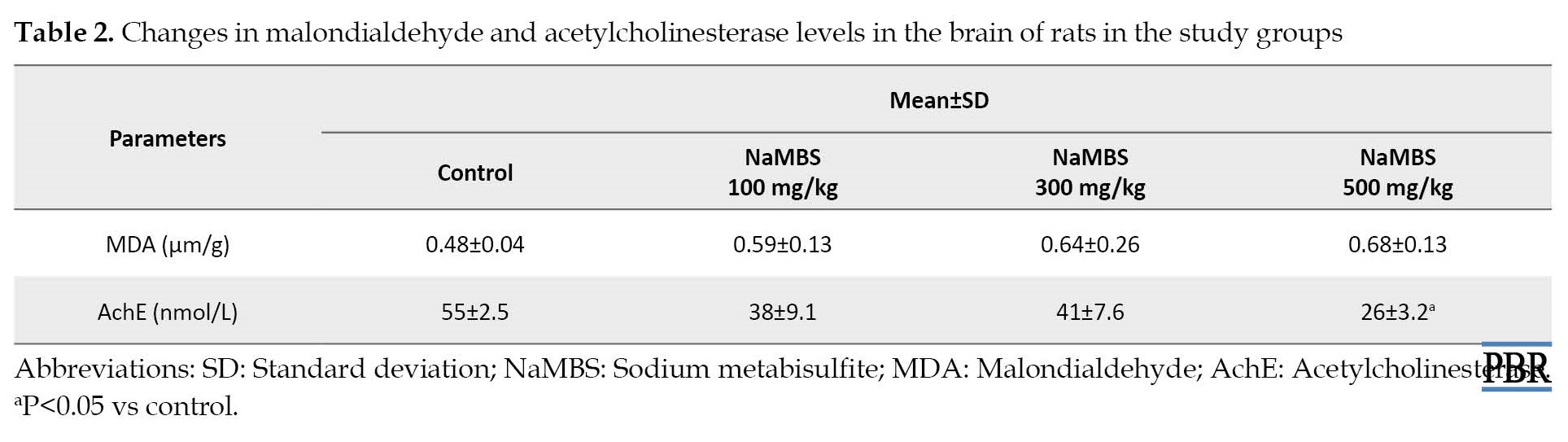
The level of AchE (
Table 2) in the group treated with NaMBS (500 mg/kg) exhibited a significant reduction (P˂0.05) compared to the control group (26±3.2 nmol/L vs 55±2.5 nmol/L). When making comparisons, no substantial variance (P>0.05) in AchE levels was observed between the groups treated with 100 mg/kg and 300 mg/kg and the control group (
Table 2).
Histopathological observations
According to
Figure 1, the H&E-stained sections of CA1 reveal closely clustered PCs with regular neuronal processes in both the control and group treated with NaMBS 100 mg/kg.
Conversely, the group treated with NaMBS 500 mg/kg presents indications of neurodegeneration, characterized by a notable presence of shrunken PCs. The assessment of neuronal cell count within CA1 (
Figure 2) demonstrates noteworthy findings.
The study group subjected to NaMBS 500 mg/kg showcases a considerably lower (P˂0.05) count of PCs (638±41) compared to the control group (750±62). Additionally, the number of PCs in the CA1 region of the study groups treated with NaMBS 100 mg/kg and NaMBS 300 mg/kg exhibit no significant variations (P>0.05) compared to the control group (
Figure 2).
According to
Figure 3, like the control group, all the treated groups exhibit appropriately organized neurons in layer II/III of the prefrontal cortex.
Additionally, the assessment of neuronal count within layer II/III (
Figure 4) indicates no statistically significant distinctions (P>0.05) between the treated groups (NaMBS 100 mg/kg, NaMBS 300 mg/kg, NaMBS 500 mg/kg) and the control group in the conducted comparisons.
According to
Figure 5, the GFAP-immunostained sections of the prefrontal cortex demonstrate no apparent immunoreactivity in both the control group and the NaMBS 100 mg/kg treated group; however, in the prefrontal cortex of the NaMBS 300 mg/kg and 500 mg/kg treated groups, there is limited immunoreactivity (indicated by yellow arrows) characterized by astrocytes with star-shaped appearances situated near neurons.
Moreover, the quantification of GFAP-immunoreactive cells in the prefrontal cortex (
Figure 6) reveals that the number of immunoreactive cells in the NaMBS 300 mg/kg and 500 mg/kg treated groups (140±11 and 151±18, respectively) was significantly higher (P˂0.05) compared to the control group (93±6).
According to
Figure 7, the GFAP-immunostained section of CA1 illustrates an absence of evident astrocyte immunoreactivity within the control group.
However, the CA1 region of the NaMBS 100 mg/kg treated group displays limited immunoreactivity (green arrows). The NaMBS 300 mg/kg and 500 mg/kg treated groups exhibit pronounced immunoreactivity (red arrows), characterized by a substantial presence of star-shaped astrocytes closely associated with pyramidal neurons. Furthermore, the quantification of GFAP-immunoreactive cells in CA1 (
Figure 8) demonstrates a significant increase (P˂0.05) in the number of immunoreactive cells across all the treated groups (48±1.5, 91±15, and 99±13) compared to the control group (6±2.6).
Discussion
Sodium metabisulfite is a commonly utilized substance for preserving and protecting food, serving as an antioxidant. Nonetheless, there have been documented instances of its negative impacts on the nervous system. The hippocampus is a critical brain region responsible for memory formation, learning, and spatial navigation [
21]. The prefrontal cortex, on the other hand, is responsible for planning, decision-making, and social behavior. Research has shown that exposure to toxins can cause cell damage, inflammation, and oxidative stress, leading to impaired function of these brain regions, such as impaired cognitive and learning function [
22].
This study has shown that NaMBS 500 mg/kg induces neurodegeneration in CA1 of the hippocampus and affects the number of neurons in the same area. NaMBS enhances sodium currents in rat hippocampal neurons, increasing their excitability and potentially leading to oxidative damage [
9]. Also, lipid peroxidation causes excess oxidative stress, which in turn causes cell death [
23]. This result indicates that exposure to this compound at 500 mg/kg leads to the progressive loss of neurons or neuronal function in the CA1 area of the hippocampus, which can subsequently result in cognitive and functional consequences associated with hippocampal dysfunction [
24]. The hippocampus plays a crucial role in memory formation and learning, so any damage or degeneration in this region can have significant cognitive and behavioral impacts.
This study has also shown that NaMBS at a dose of 500 mg/kg does not negatively impact the neurons or the overall cellular structure (cytoarchitecture) of the prefrontal cortex. The degeneration of hippocampal neurons under the influence of NaMBS and the lack of noticeable impact in the prefrontal cortex could be due to differences in the expression of sodium channels, the brain’s structural redundancy, and its ability to compensate for damage through biochemical and morphological plasticity [
9,
25]. Also, research has shown that exposure to the same toxic substance can lead to different patterns of damage or outcomes in other brain areas [
25]. In this study, NaMBS elicits increased GFAP-immunoreactivity within the prefrontal cortex. Increased GFAP-immunoreactivity often indicates the activation or response of astrocytes to certain types of brain injury, inflammation, or other neurological changes [
26]. The absence of visible effects on neurons and cytoarchitecture, combined with increased immunoreactivity, could imply that the impact of NaMBS on the prefrontal cortex is more subtle and might involve biochemical or molecular changes rather than gross structural alterations.
The Y-Maze spontaneous alternation test is based on the innate tendency of rodents to explore the maze, entering each arm consecutively [
15]. This function relies on the ability of their hippocampal cells to fire in complex bursts when they move through specific locations in the environment. This hippocampal-dependent memory is called spatial memory, a form of episodic memory [
21]. This study shows no statistical significance in a complete alternation of the rats in the treatment group that received NaMBS compared to rats in the control group. Considering that the Y-Maze Spontaneous alternation test is a test for hippocampal-dependent memory in rodents, the result indicates that hippocampal-dependent memory is not impaired in the NaMBS-exposed group. The preservation of intact memory despite observed damage to neurons in the CA1 area of the hippocampus in this study may be because of the brain’s ability to reorganize, rely on alternative pathways, and adapt to damage [
27]. Also, compensatory mechanisms, redundancy in neural circuits, and neural plasticity could be reasons behind this observation.
This study shows that the administration of NaMBS at the doses utilized will not significantly affect the MDA concentration. Accordingly, the compound did not induce significant oxidative stress or lipid peroxidation at these specific doses, which is a positive finding as elevated MDA levels are generally associated with cellular damage and dysfunction [
28]. While the study did not reveal a significant concentration of MDA, lipid peroxidation may remain elevated. MDA is not a direct indicator of lipid peroxidation and can be influenced by various factors [
29]. This potential discrepancy could account for the observed neuronal degeneration in the study.
AchE is a critical enzyme in regulating the neurotransmitter acetylcholine levels, essential for proper nervous system functioning, muscle contraction, and cognitive processes [
30]. The inhibition of this enzyme may lead to decreased breakdown and subsequent accumulation of acetylcholine, which could result in excitotoxicity and impaired memory [
31]. In this study, the level of AchE in the group treated with NaMBS (500 mg/kg) exhibited a significant reduction compared to the control group. A decrease in the level of AchE suggests that the enzyme’s activity is diminished. This could result in an accumulation of acetylcholine, the neurotransmitter responsible for transmitting signals between nerve cells. This reduction in AchE activity could have implications for neurological functions, including excitotoxicity and cognitive activities. Despite the possible accumulation of acetylcholine in the brains of rats in the study, learning and memory activities were unaffected. This is because of the debatable role being played by acetylcholine, as it is believed to be more involved in attention processing than learning and memory [
32].
Conclusion
In conclusion, this study sheds light on the complex effects of NaMBS on the nervous system. While it induces neurodegeneration in the hippocampus at a dose of 500 mg/kg, the prefrontal cortex seems resilient to structural changes. Spatial memory remains unaffected, aligned with histological and biochemical analyses. The reduction in acetylcholinesterase activity raises concerns about potential cognitive implications, emphasizing the need for further investigation into the intricate mechanisms underlying NaMBS’s impact on neurological function.
Ethical Considerations
Compliance with ethical guidelines
Animals were handled according to the Research Ethics of Adeleke University Ede, Osun State, Nigeria (No.: 00656) in line with the National Institute of Health Guidelines for Care and Use of Laboratory Animals (Publication 1985).
Funding
This research did not receive any grant from funding agencies in the public, commercial, or non-profit sectors.
Authors' contributions
Conceptualization and Supervision: Nathaniel Ohiemi Amedu; Methodology: Nathaniel Ohiemi Amedu; Investigation and original draft: Favour Wilcox and Habeeb Abdur-Rahman; Review and editing of final draft: All authors; Data collection and Analysis: Nathaniel Ohiemi Amedu, Patrick Abolarin, Favour Wilcox, and Habeeb Abdur-Rahman; Funding acquisition and resources: Nathaniel Ohiemi Amedu and Favour Wilcox.
Conflict of interest
The authors declared no conflict of interest.
Acknowledgments
The authors take this opportunity to thank Dan Ajibade and Ezra Lambe for their technical support.
References
- Noorafshan A, Asadi-Golshan R, Abdollahifar MA, Karbalay-Doust S. Protective role of curcumin against sulfite-induced structural changes in rats' medial prefrontal cortex. Nutr Neurosci. 2015; 18(6):248-55. [DOI:10.1179/1476830514Y.0000000123] [PMID]
- Sadowska B, Sztormowska M, Gawinowska M, Chelminska M. Sodium metabisulfite hypersensitivity in urticaria. Our Dermatol Online. 2021; 12(2):106-12. [DOI:10.7241/ourd.20212.2]
- Lavoie JC, Lachance C, Chessex P. Antiperoxide activity of sodium metabisulfite. A double-edged sword. Biochem Pharmacol. 1994; 47(5):871-6. [DOI:10.1016/0006-2952(94)90487-1] [PMID]
- Mishra LK, Yadav B. Assessing the cytotoxic and genotoxic effects of sodium metabisulphite: A food preservative. Vegetos. 2011; 24(1):32-7. [Link]
- Alimohammadi A, Moosavy MH, Amin Doustvandi M, Baradaran B, Amini M, Mokhtarzadeh A, et al. Sodium metabisulfite as a cytotoxic food additive induces apoptosis in HFFF2 cells. Food Chem. 2021; 358:129910. [DOI:10.1016/j.foodchem.2021.129910] [PMID]
- Kadi FZ, Bénali AI, Bénali M, Belbraouet S. Effect of sodium metabisulphite on blood metabolic status of wistar rats. Food Nutr Sci. 2014; 5(15):1529-37. [DOI:10.4236/fns.2014.515165]
- Shekarforoush S, Ebrahimi P, Fathabad AA, Farzanfar E. Effect of sodium metabisulfite on oxidative stress and lipid peroxidation biomarkers. Curr Nutr Food Sci. 2020; 16(1):114-17. [DOI:10.2174/1573401314666181024130333]
- Ercan S, Oztürk N, Celik-Ozenci C, Gungor NE, Yargicoglu P. Sodium metabisulfite induces lipid peroxidation and apoptosis in rat gastric tissue. Toxicol Ind Health. 2010; 26(7):425-31. [DOI:10.1177/0748233710369665] [PMID]
- Meng Z, Nie A. Effects of sodium metabisulfite on potassium currents in acutely isolated CA1 pyramidal neurons of rat hippocampus. Food Chem Toxicol. 2005; 43(2):225-32. [DOI:10.1016/j.fct.2004.09.008] [PMID]
- Ozkan A, Parlak H, Agar A, Ozsoy O, Tanriover G, Dilmac S, et al. The effect of sodium metabisulphite on apoptosis in the experimental model of parkinson’s disease. Curr Nutr Food Sci. 2020; 16(3):296-305. [DOI:10.2174/1573401314666180503153444]
- Parlak H, Ozkan A, Ozsoy O, Tanriover G, Dilmac S, Turgut E, et al. The effect of sodium metabisulphite on locomotor activity in the experimental model of parkinson’s disease: The role of cyclooxygenase. J Nutr Food Sci. 2018; 8(3):1000694. [DOI:10.4172/2155-9600.1000694]
- Meng Z, Nie A. Enhancement of sodium metabisulfite on sodium currents in acutely isolated rat hippocampal CA1 neurons. Environ Toxicol Pharmacol. 2005; 20(1):35-41. [DOI:10.1016/j.etap.2004.10.003] [PMID]
- Thierry AM, Gioanni Y, Dégénétais E, Glowinski J. Hippocampo-prefrontal cortex pathway: Anatomical and electrophysiological characteristics. Hippocampus. 2000; 10(4):411-9. [DOI:10.1002/1098-1063(2000)10:43.0.CO;2-A] [PMID]
- Lai MC, Hung TY, Lin KM, Sung PS, Wu SJ, Yang CS, et al. Sodium metabisulfite: Effects on ionic currents and excitotoxicity. Neurotox Res. 2018; 34(1):1-15. [DOI:10.1007/s12640-017-9844-4] [PMID]
- Kraeuter AK, Guest PC, Sarnyai Z. The Y-maze for assessment of spatial working and reference memory in mice. Methods Mol Biol. 2019; 1916:105-11. [DOI:10.1007/978-1-4939-8994-2_10] [PMID]
- Gage GJ, Kipke DR, Shain W. Whole animal perfusion fixation for rodents. J Vis Exp. 2012; 65:e3564. [DOI:10.3791/3564]
- Ellman GL, Courtney KD, Andres V Jr, Feather-Stone RM. A new and rapid colorimetric determination of acetylcholinesterase activity. Biochem Pharmacol. 1961; 7:88-95. [DOI:10.1016/0006-2952(61)90145-9] [PMID]
- Bancroft JD, Gamble M. Theory and practice of histological techniques. London: Churchill Livingstone; 2008. [Link]
- Amedu NO, Obu MO. Neuroprotective effects of vitexin and cajanus cajan extract against Pb-induced neurotoxicity in wistar rats. Pharm Biomed Res. 2022; 8(4):291-300. [DOI:10.32598/PBR.8.4.1065.1]
- Amedu NO, Obu MO. Atrazine-induced hippocampal degeneration and behavioral deficits in wistar rats: Mitigative role of avocado oil. Iran J Toxicol. 2022; 16(3):211-20. [DOI:10.32598/IJT.16.3.949.2]
- Kühn S, Gallinat J. Segregating cognitive functions within hippocampal formation: A quantitative meta-analysis on spatial navigation and episodic memory. Hum Brain Mapp. 2014; 35(4):1129-42. [DOI:10.1002/hbm.22239] [PMID] [PMCID]
- Genuis SJ, Kelln KL. Toxicant exposure and bioaccumulation: A common and potentially reversible cause of cognitive dysfunction and dementia. Behav Neurol. 2015; 2015:620143. [DOI:10.1155/2015/620143] [PMID] [PMCID]
- Amedu NO, Omotoso GO. Influence of vitexin on ataxia-like condition initiated by lead exposure in mice. Toxicol Environ Health Sci. 2020; 12:305-13. [DOI:10.1007/s13530-020-00041-x]
- Lieberman JA, Girgis RR, Brucato G, Moore H, Provenzano F, Kegeles L, et al. Hippocampal dysfunction in the pathophysiology of schizophrenia: A selective review and hypothesis for early detection and intervention. Mol Psychiatry. 2018; 23(8):1764-72. [DOI:10.1038/MP.2017.249] [PMID] [PMCID]
- Bjornaes S, Naalsund LU. Biochemical changes in different brain areas after toluene inhalation. Toxicology. 1988; 49(2-3):367-74. [DOI:10.1016/0300-483X(88)90020-0] [PMID]
- Hajós F. Changes in glial fibrillary acidic protein (GFAP) immonureactivity reflect neuronal states. Neurochem Res. 2008; 33(8):1643-50. [DOI:10.1007/S11064-008-9745-2] [PMID]
- Toricelli M, Pereira AAR, Souza Abrao G, Malerba HN, Maia J, Buck HS, et al. Mechanisms of neuroplasticity and brain degeneration: Strategies for protection during the aging process. Neural Regen Res. 2021; 16(1):58-67. [DOI:10.4103/1673-5374.286952] [PMID] [PMCID]
- Singh Z, Karthigesu IP, Singh P, Rupinder KA. Use of malondialdehyde as a biomarker for assessing oxidative stress in different disease pathologies: A review. Iran J Public Health. 2014; 43(Supple 3):7-16. [Link]
- Janero DR. Malondialdehyde and thiobarbituric acid-reactivity as diagnostic indices of lipid peroxidation and peroxidative tissue injury. Free Radic Biol Med. 1990; 9(6):515-40. [DOI:10.1016/0891-5849(90)90131-2] [PMID]
- Härtl R, Gleinich A, Zimmermann M. Dramatic increase in readthrough acetylcholinesterase in a cellular model of oxidative stress. J Neurochem. 2011; 116(6):1088-96. [DOI:10.1111/J.1471-4159.2010.07164.X] [PMID]
- Colović MB, Krstić DZ, Lazarević-Pašti TD, Bondžić AM, Vasić VM. Acetylcholinesterase inhibitors: Pharmacology and toxicology. Curr Neuropharmacol. 2013; 11(3):315-35. [DOI:10.2174/1570159X11311030006] [PMID] [PMCID]
- Blokland A. Acetylcholine: A neurotransmitter for learning and memory? Brain Res Brain Res Rev. 1995; 21(3):285-300. [DOI:10.1016/0165-0173(95)00016-X] [PMID]