Introduction
Trbinafine hydrochloride is an artificial allylamine antifungal that treats mold infections. It accumulates in fatty tissues, the skin, and nails and has a significant lipophilic inclination [
1]. Like other allylamines, terbinafine inhibits squalene epoxidase, an enzyme involved in the pathway leading to the development of the fungal cell wall, hence preventing the production of ergosterol. Terbinafine hydrochloride is recommended for treating fungal skin and nail infections caused by Trichophyton species, Microsporum canis, Epidermophyton floccosum, and Tinea species. Additionally, cutaneous yeast infections caused by Candida species and Malassezia furfur can be treated with terbinafine hydrochloride [
2].
Terbinafine inhibits the enzyme squalene monooxygenase, commonly referred to as squalene epoxidase, which stops squalene from being converted to 2, 3-oxydosqualene, the first step in the formation of ergosterol [
3,
4]. Squalene accumulates due to this inhibition, and less ergosterol than would normally be integrated into the cell wall is created. Squalene-containing cytoplasmic vesicles in large quantities may remove other lipids from the cell wall, causing further harm [
5].
Medication delivery is heavily employed in nanotechnology for passive and active targeting through various administration techniques. Colloidal particulate systems with diameters ranging from 10 to 1000 nm are known as nanoparticles. In response to the drawbacks of the first generation of lipid nanoparticles, nanostructured lipid carriers (NLCs) are emerging as the second generation [
6]. NLCs are made with emulsifiers, suitable liquid and solid lipids, and biodegradable lipids. When liquid lipids, such as oil, are introduced to solid lipids, they develop structural flaws that result in a less ordered crystalline arrangement that offers a high drug load and stops drug leakage [
7].
These days, lipid-based drug delivery techniques are commonly employed due to their capacity to increase the solubility and bioavailability of medications that are either lipophilic or poorly soluble in water. The development of an improved generation of lipid nanoparticles known as NLCs has solved the problems with conventional lipid-based formulations and solid lipid nanoparticles [
8]. NLCs have become a feasible carrier system for the oral, parenteral, ocular, pulmonary, topical, and transdermal delivery of pharmaceuticals. NLCs have recently been utilized in delivering brain targeting, gene therapy, chemotherapy, and cosmeceuticals and nutraceuticals [
9].
Hydrogels are three-dimensional cross-linked polymer networks that absorb a lot of water when immersed in an aqueous solution. They have many properties of solids and liquids because of this unique structure, such as a large amount of free water and possibly soluble molecules that can seep into and out of the gels. These polymers have good swelling qualities and are biocompatible, biodegradable, and non-toxic due to their highly water-holding (often between 30% and 90%) three-dimensional cross-linked hydrophilic networks. Scientists all around the world are currently very interested in hydrogel-based wound dressings because of their wide range of biocompatible matrices and physiologically active components, which include neutralized polyacrylic acid, chitosan, cellulose, starch, and alginate [
10].
Hydrogels can be made from two different types of polymers: Synthetic and natural. Most synthetic polymers are chemicals that dissolve, spread, or swell when placed in water. They modify the physical properties of aqueous solutions through gelatinization, thickening, or stabilization of emulsions [
11]. These polymers usually consist of blocks of units or repeating units with hydrophilic groups (nonionic, anionic, cationic, or amphoteric) integrated into the polymer chains or attached to them as substituents. The synthetic polymer poly (ethylene glycol) or PEG is highly soluble in organic solvents. PEG's aqueous solubility and low intrinsic toxicity make it appropriate for biological applications. PEG's strong hydrophilic characteristics increase its solubility when paired with hydrophobic drugs or carriers. It improves pharmaceuticals' chemical and physical stability and keeps them from clumping together in vivo and during storage [
12].
In addition to preparing and optimizing terbinafine hydrochloride-loaded (TH) NLC for topical administration, the current work aimed to assess the produced formulation for comparison of the drug release and permeation between optimized terbinafine hydrochloride loaded NLC (TH_NLC)opt formulation and TH_NLCopt-based gel formulation and select which one was the best for topical drug delivery.
Materials and Methods
Study materials
Terbinafine hydrochloride was a gift sample from Macleods Pharmaceuticals Ltd (Andheri East, Mumbai). Stearic acid was received from LOBA CHEMIE PVT.Ltd (Mumbai, Maharashtra), and olive oil of BERTOLLI was purchased locally. The suppliers of stearic acid and carbopol 934 P were provided by Loba Chemie Pvt. Ltd., Mumbai, Maharashtra 400005. Tween 80 was received from Merck Specialities Private Limited (Shiv Sagar Estate ‘A,’ Mumbai- 400 018) and selected as a surfactant. Triethanolamine of Loba Chemie Pvt. Ltd., Mumbai, Maharashtra 400005 was chosen as a gel neutralizing agent, and methanol of Merck Specialities Private Limited (Shiv Sagar Estate ‘A,’ Mumbai- 400 018) was selected as a solvent for dissolving NLC product. Double distilled water was prepared in the Laboratory of Guru Nanak Institute of Pharmaceutical Science and Technology (Panihati, Sodepur, Kolkata-700 114).
Study methods
Compatibility study of the materials
Fourier-transform infrared spectroscopy (FTIR) analysis was used in this work to determine the drug’s (terbinafine hydrochloride) compatibility with the other materials (stearic acid, olive oil, carbopol 934 P, etc.) [
13]. Terbinafine hydrochloride, stearic acid, olive oil, and their physical mixtures were all subjected to infrared transmission properties analyses using an FTIR spectrophotometer (Perkin Elmer Spectrum Two). The KBr pellet method was employed to create the sample, and the FTIR spectra were recorded using an FTIR analyzer. A thin pellet was compressed after fine powder grinding, and a mass ratio 1:100 was used to combine the samples with anhydrous KBr. The scanning range was 4000-400 cm-1, and the pressure was 5.5 metric tonnes for three minutes under a hydraulic press [
14].
Experimental design of NLC
In the current study, an optimization study was conducted using a central composite face-cantered (α=1) design with two components and thirteen runs using Design-Expert® software (version 7.1.5) [
15]. The independent variables, homogenizer speed and liquid lipid amount, were selected and set at high and low values, respectively.
Table 1 presents the coded values for several variables.
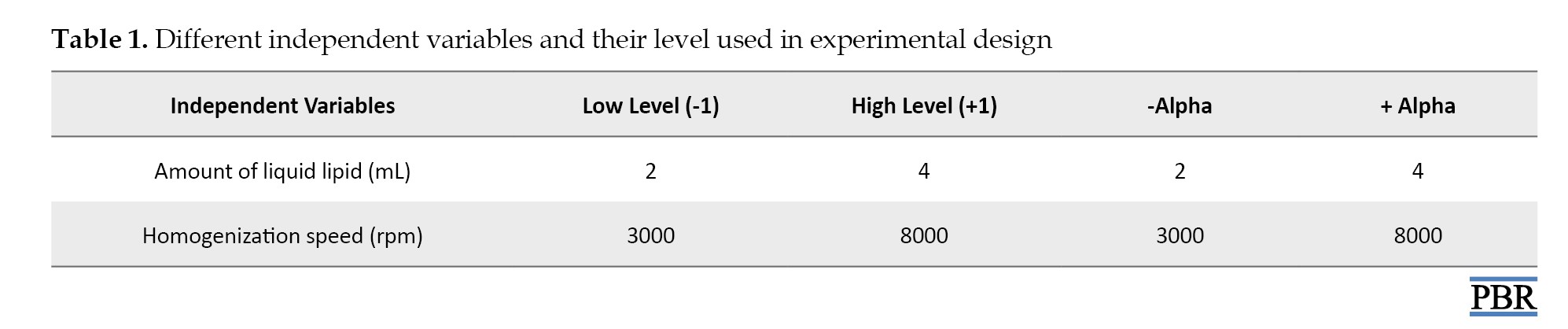
The strategy produced thirteen NLC formulations, which were subsequently evaluated for the response criteria of entrapment efficacy (R2) and particle size (R1) [
16].
Table 2 listed 13 NLC formulations in accordance with the central composite design.
Preparation of TH NLC
First, a magnetic stirrer (REMI 1MLH) from REMI Elektrotechnik Ltd. (Vasai, India) was used to constantly stir stearic acid, a solid lipid, and olive oil, a liquid lipid, in a heated environment (between 60 °C and 70 °C). Terbinafine hydrochloride was also produced in an aqueous form and heated to the same temperature. After that, using a magnetic stirrer (REMI 1MLH) from REMI ELEKTROTECHNIK Ltd. (Vasai, India) and a surfactant (tween 80), the aqueous and lipid phases were combined at a heated temperature (60 °C to 70 °C). The mixture was run for 20–30 minutes at 3000-8000 rpm through a homogenizer (REMIMOTOR) owned by REMI ELEKTROTECHNIK Ltd. (Vasai, India). The procedure was carried out while the temperature remained high. Using an Ultrasonic Sonicator from LABMAN SCIENTIFIC INSTRUMENT PVT. Ltd. (Chennai-600118, India), the mixture was sonicated for 20–30 minutes and left to stand at room temperature for 48 hours [
17]. The formulations were then lyophilized using OPTICS TECHNOLOGY’s Lyophilizer (Delhi-34, India).
Table 3 provides the composition of the various NLC formulations [
18].
Characterization of TH_NLC formulation
Physical appearance
Visual inspection assessed the prepared TH_NLCs’ physical appearance [
19].
Particle
Using a Zetasizer software, version 7.11 (Malvern Instruments Ltd, UK), dynamic light scattering (DLS) was used to determine the particle size of the NLC formulations at a temperature of 25 ºC. Merck Life Science Pvt. Ltd.’s (Mumbai, India) methanol was used to dissolve the NLC formulations. They were examined for particle size after being diluted 100 times with methanol [
20].
Morphological analysis
The TH_NLC 1 formulation underwent morphological examination using scanning electron microscopy (SEM) (ZEISS EVO 18, CARL ZEISS MICROSCOPY, PENTA FET X 3). Scanner microscopy was used to check the particle’s size and shape. The NLC was lyophilized and then subjected to SEM examination. The TH_NLC formulation was dissolved in methanol purchased from Merck Life Science Pvt. Ltd (Mumbai, India) will prepare the sample. A grid was covered with a few drops of dispersion and left to dry. The samples were photographed once they had completely dried. It was found that the TH_NLC formulation’s particle sizes varied from less than 100 to 200 nm [
21].
% entrapment efficiency
Using a cooling-centrifugation process, the EE% was found. One gram of TH_NLC was dissolved in 10 mm of pH-7.4 phosphate [
22]. With a cooling centrifuge from REMI ELEKTROTECHNIK Ltd. (Vasai, India), the dispersion was cold centrifuged (5-8 ºC) for 20 minutes at 12000 rpm. The filter paper filters the liquid supernatant (Mess No. 11 µm). After that, the filtrate was diluted, and a UV spectrophotometer (JASCO, V630 Spectrophotometer) at 285 nm wavelength was used to measure the optical density. From there, the amount of drug in the supernatant liquid was computed.
Equation 1 was used to determine the EE% [
23].
Optimization of the formulation
TH_NLC formulations were optimized using Design-Expert® software regarding response, including particle size and entrapment efficiency. Initially, the analysis of the quadratic model was chosen. The model was then examined using the analysis of variance (ANOVA) and R2 tests. Particle size and entrapment efficiency were shown to be affected by the independent parameters independently (A or B), jointly (AB), and quadratically (A2, B2). Three-dimensional response surface graphs, in addition to a polynomial equation that showed the design’s result, three-dimensional response graphs were created. Applying numerical optimization, optimal solutions were identified as optimized products. From these, one formulation was chosen for further assessment of the optimized product due to its decreased particle size and improved entrapment efficiency [
16,
24].
Preparation of TH_NLCopt gel
Using carbopol 934 of Loba Chemie Pvt Ltd. (107, Wodehouse Rd, Colaba, Mumbai, Maharashtra 400005) as a gelling agent, the point prediction-based optimized TH_NLCopt was converted into a gel. The prepared TH_NLCopt dispersion was added in the presence of Tween 80 while being continuously stirred with a magnetic stirrer (REMI 1MLH) from REMI ELEKTROTECHNIK Ltd. (Vasai, India) to the carpool (1% w/v) dispersed in distilled water overnight to create uniform dispersion. The pH of the gel system was finally neutralized by adding triethanolamine of Loba Chemie Pvt Ltd. (107, Wodehouse Rd, Colaba, Mumbai, Maharashtra 400005) [
25].
Evaluation of TH_NLCopt gel
A digital pH meter (Systronics 335, India Ltd.) was used to measure the TH_NLCopt gel’s pH at room temperature [
26] NLCs. Gel spreadability testing is one of the most essential steps in determining how well gel flows. The measured amount of TH_NLCopt gel (5 g) was placed on the glass slide using the pre-marked diameter of 5 cm before being covered with another slide. The second glass slide had a weight (20 g) connected to it, and the time it took the gel to travel 5 cm was recorded [
27]. The viscosity of the gel sample was measured at room temperature using a Brookfield viscometer. At 20 rpm, viscosity was measured using spindle number S-64 [
28]. Visual examination of the gel formulation allows for assessing the homogeneity of TH_NLCopt gel [
29]. The extrudability test aimed to determine how well gel extruded from a collapsible tube under continual weight application. Ten grams of TH_NLCopt gel were pressed out of the collapsible tube with a constant load of 100 g. The extruded gel was collected and weighed once the cap was removed. The extruded gel percentage >90% was computed (good extrudability, greater than 80% good extrudability, over 70% fair extrudability) [
30] ”ISSN”:”19994923”,”abstract”:”Superficial fungal diseases of the skin and nails are an increasingly common occurrence globally, requiring effective topical treatment to avoid systemic adverse effects. Polymeric nanoparticles have demonstrated sustained and effective drug delivery in a variety of topical formulations. The aim of this project was to develop polymeric antifungal nanospheres containing terbinafine hydrochloride (TBH. The use of a cooling-centrifugation process discovered the drug content. Ten milliliters of pH-7.4 phosphate buffer was used to dissolve 1 g of TH-NLCopt gel. The dispersion was cold centrifuged at a temperature of 5-8 ºC using a cooling centrifuge from REMI ELEKTROTECHNIK LTD. (Vasai, India), running at 12000 rpm for 20 minutes. The supernatant liquid was separated from the dispersion using filter paper with a mesh size of 11 µm. If necessary, the liquid supernatant was further diluted. Once the filtrate had been diluted, the optical density was measured with a UV spectrophotometer (JASCO, V630 Spectrophotometer) set to 285 nm wavelength. Using these data, the drug concentration in the liquid supernatant was then calculated [
26].
In vitro drug release study using dialysis membrane
Utilizing a dialysis membrane 50 (Hi-Media) with a receptor compartment capacity of 45 mL and a cross-sectional area of 0.785 cm2 was accomplished by using a Franz diffusion cell apparatus. The membrane was treated with the synthesized TH_NLCopt and TH_NLCopt gel formulation [
31]. The mended membrane was then positioned in the donor chamber to face the receiving compartment. The receiver compartment was filled with 7.4 pH PBS buffer. Magnetic beads constantly stir the receptor solution and maintain a temperature of 32±0.5 ºC. The drugs were assessed after the samples were taken at 15, 30, 60, 90, 120, 180, 240, 300, 360, and 420 minutes. Then, 4 mL of receptor solution that had been removed was replaced with a fresh buffer that had a pH of 7.4. The materials were examined for drug content using a UV-VIS spectrophotometer (JASCO, V630 Spectrophotometer) set at 285 nm [
32] drug dissolution/diffusion and polymeric combination. For the preparation of controlled release dosage form, hydrophilic, swellable polymers in optimum combination are essential. The major objective of the current study is to prepare Amoxicillin trihydrate-loaded bucco-matrix tablets by direct compression technique and to study the effect of ratio of HPMCK100M and HPMCK15M used in the formulation on the basic properties and on drugrelease and permeation kinetics. The tablets offered satisfactory physicochemical results. The buccal strength, detachment force and bond strength of the tablets were good enough to hold the tablets in the buccal region. The drug release data generated during in vitro drug release study of bucco-matrix tablets in phosphate buffer pH 6.8 were evaluated by zero-order, first-order, Higuchi, Korsmeyer - Peppas, and Kopcha models. Release exponent (n. The computation was carried out using the slope discovered from the terbinafine hydrochloride standard curve in pH -7.4 phosphate buffer. The following equation yields the slope (0.0074). The regression coefficient is 0.9997, and y=0.0074x [
33].
Ex vivo permeation study using goat abdominal skin
Goat abdomen skin that had recently been taken from an abattoir was employed in this investigation. It was hydrated in a pH 7.4 PBS buffer for one hour [
17]. As previously stated, the ex vivo skin penetration investigation used a 45 mL Franz diffusion cell. The goat faced the donor compartment when 1 g of the TH_NLCopt and TH_NLCopt gel formulation was applied to the stratum corneum of its abdomen skin. After that, the donner compartment was placed so that the skin was deep into the upper half of the buffer solution in the diffusion cell’s receiver compartment, which held pH 7.4 PBS buffer. The receptor solution was continually stirred with a magnetic bead while maintaining a temperature of 32±0.5 ºC. The drug content was assessed after the samples were taken out at 15, 30, 60, 90, 120, 180, 240, 300, 360, and 420 minutes. Then, 4 mL of receptor solution that had been removed was replaced with a fresh buffer that had a pH of 7.4. To find out if any medications were present, the samples were exposed to UV-visible spectroscopy (JASCO, V630 Spectrophotometer) at a wavelength of 285 nm [
34]. The calculation was carried out in a pH-7.4 phosphate buffer using the slope of the terbinafine hydrochloride standard curve. The percentage of the retained drug to the penetrated drug was determined using the UV spectrophotometric data [
31].
Statistical method
As a statistical method, ANOVA was used to detect any non-significant components in the study.
Results
Compatibility study of the materials
Infrared experiments investigated potential physical or chemical interactions between terbinafine hydrochloride and its excipients (olive oil and stearic acid).
Figure 1 displays the resulting IR spectrum.

The FTIR spectra of terbinafine hydrochloride showed that the C-C in the ring and Ar2NH groups were all visible. The stretch bands for the Ar-C and Ar-N groups were located at 1490 cm-1 and 1326 cm-1, respectively. The stretch band for RCOOH, R – OH, RCO – O-, and C=C-CO-OH group of carbopol 934 P were located at 1015 cm-1, 1762 cm-1, 1600 cm-1, and 3333 cm-1, respectively. The stretch band for RCOOH, RCOOH, and RCH2CH3 group of stearic acid were located at 1142 cm-1, 2989 cm-1, and 2989 cm-1, respectively. C-C in ring, Ar2NH, RCOOH, RCOOR1, C=C-CO-OH, R – OH, and RCO – O- exhibited stretched bands at 1324 cm-1, 1131 cm-1, 1236 cm-1, 3331 cm-1, 1760 cm-1, and 1605 cm-1, respectively, which were comparable to the physical composition of terbinafine hydrochloride, stearic acid, olive oil and carbopol 934 P. These typical transmission bands showed no physical or chemical interaction between the medication, olive oil, stearic acid, and carbopol 934 P. The excipients and the medication worked flawlessly together.
Characterization of TH_NLC formulation
Physical appearance
After producing the product (nanostructured lipid carriers), the homogeneity, texture, and condition of the TH_NLC formulations are appropriate for additional investigation.
Particle size
The preparation of the terbinafine hydrochloride-loaded NLCs (TH_NLC) involved varying the volumes of liquid and solid lipids and the speed at which they were homogenized. The findings of examining the particle size of the synthesized TH_NLC formulations are displayed in
Figure 2.
Particle sizes of TH_NLCs ranged from 17.57 (TH_NLC6) to 329.4 nm (TH_NLC3). The drug’s particle size significantly impacts its capacity to penetrate the skin and stay in the epidermis.
Morphological analysis
Figure 3 displays the SEM pictures of the TH_NLC1 formulation at various magnifications.
The image shows the TH_NLC1 formulation’s large particle distribution. The findings verified the TH_NLC1 formulation’s surface shape, and the particle size was between 52 and 176 nm.
% entrapment efficiency
The entrapment effectiveness of the prepared TH_NLCs was calculated using the indirect technique (
Table 4).

It was discovered that the TH_NLC formulations’ entrapment efficiencies ranged from 60.94% to 98%. The entrapment efficiencies of formulations of TH_NLC2 to TH_NLC8 were 98% and 60.946%. Because liquid lipid in NLCs introduces defects into the order crystal structure and increase the space available to retain more medication, it affects loading and entrapment.
Optimization of the formulation
Statistical method
The NLC formulations were optimized using a two-factor, three-level, face-centered central composite formulation design approach to get the optimal composition with the lowest particle size and the highest entrapment effectiveness. The design exhibited 13 formulation compositions with 5 common compositions to check for any faults in the findings. The information on particle size (R1) and entrapment efficiency (R2) was considered to interpret the results. The program generated predicted values, polynomial equations, and 3D response surface graphs to evaluate the impact of independent factors on dependent components. The outcome was closer to the predicted value (
Table 5) when the expected value generated by the program was compared to the actual particle size and entrapment efficiency.

The best-fit model (R2) for all responses was the quadratic model with the highest correlation coefficient value (
Table 6).
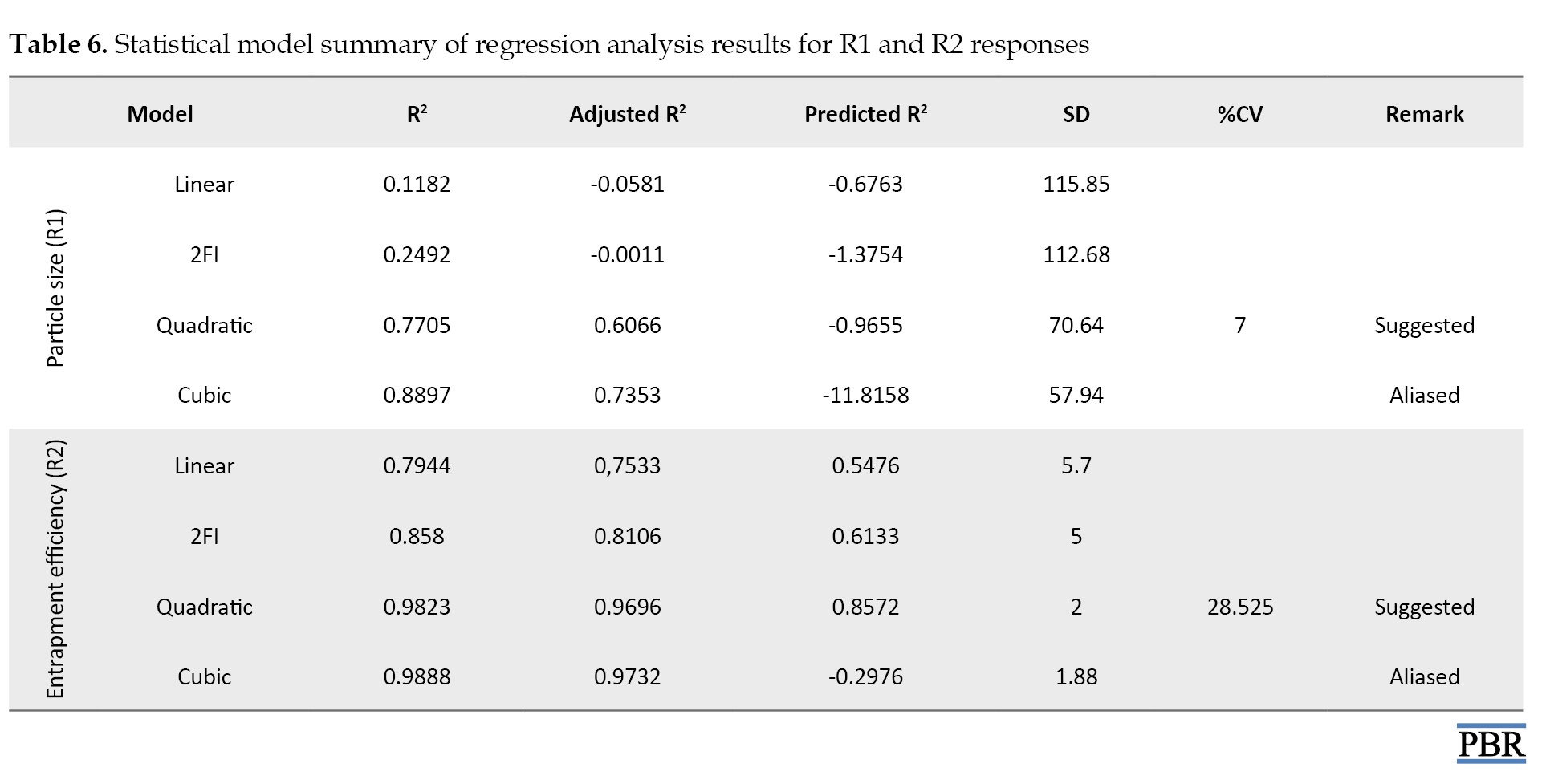
The quadratic response was the best option for optimization since the selected independent variable affected the dependent variables both singly and collectively.
Regression model analysis
According to
Table 6, a negative predicted R2 for particle size indicates that the overall mean predicts the reaction more accurately than the current model. The adequate precision measures the signal-to-noise ratio. Ideally, the ratio should be higher than 4. A ratio of 7.000 in this investigation denotes a sufficient signal. The design area can be navigated with the help of this model. Regarding entrapment efficiency, the adjusted R2 of 0.9696 and the predicted R2 of 0.8572 are reasonably in agreement. The adequate precision measures the signal-to-noise ratio. Ideally, the ratio should be higher than 4. A sufficient signal in this investigation is indicated by a ratio of 28.525. The design area can be navigated with the help of this model, named the quadratic model.
The ANOVA result was deemed significant since the F value showed a value greater than 4. Residual values were found to support the optimization, and the degree of lack of fit was deemed negligible (
Table 7).

The model seemed significant because the P<0.05, precisely 0.0335 for particle size and <0.0001 for Entrapment efficiency. A calculation of the software’s accuracy as a percentage prediction was done. The interaction between independent and dependent variables is encouraged by the polynomial equation’s positive sign, whereas the reverse relationship is shown by its negative value.
Effect of independent variables on particle size (R1) and entrapment efficiency (R2)
Equations 2 and
3 were obtained after analyzing the results of particle size and entrapment efficiency, respectively, describing the relationship between independent variables, particle size, and entrapment efficiency. The results of the particle size (R1) and entrapment efficiency (R2) were analyzed using ANOVA and the R2 test. The relationship between the independent variable and the responses and the interaction between the independent variables are described by the 3D response curve and counterplot, shown in
Figure 4.
2. R1=+307.88+(34.10×A)-(42.85×B)+(70.58×A×B)–(100.43×A2)–(87.98×B2)
3. R2=+85.70+(1.68×A)+(14.38×B)–(5.01×A×B)+ (4.89×A2)–(8.22×B2)
Particle size was found to be positively influenced by the amount of liquid lipid (A), as indicated by
Equation 2. According to the Equation, particle size rose as liquid lipid content decreased, most likely because homogenization speeds varied. It was also discovered that homogenization speed negatively impacted particle size (B). According to the equation, increasing the homogenization speed resulted in smaller particles. The breaking and decrease of particle size occurs at high homogenization speeds because of the tremendous force generated. Particle size was positively impacted by the mixture of homogenization speed (AB) and liquid lipid quantity. The formula demonstrates that as homogenization speed and liquid lipid amount were raised, so was particle size. This is because of the low surfactant content, which causes the lipid particles to aggregate quickly.
Based on
Equation 3, entrapment efficiency was positively impacted by the quantity of liquid lipid (A). According to the Equation, entrapment efficiency rose, as did liquid lipid content. This is because liquid lipid was present; when solid lipid ensnared the oil droplets, the drug encapsulation void grew, increasing entrapment’s effectiveness. Additionally, it was discovered that entrapment efficiency was positively impacted by homogenization speed (B). According to the equation, increasing the homogenization speed enhanced entrapment efficiency. The high shear stress resulting from the fast homogenization speed is the cause. Entrapment efficiency was adversely affected by the combined quantity of liquid lipid and homogenization speed (AB). According to the equation, the liquid lipid significantly impacted entrapment efficiency.
Point prediction
The formulation was further enhanced by the point prediction approach developed by software. The point prediction was achieved by making extremely tiny changes to the values of the independent factors to get a closer and more precise value of those parameters and their influence on the replies. This step’s main objective is to create a model with more precise results. The composition of the optimized formulation (TH_NLCopt) is as follows: 4 mL of liquid lipids and 3000 rpm for homogenization. The compound showed a 134 nm particle size and a 75% entrapment efficiency. It was found that there was a very close relationship between the expected and actual values. It was found that the anticipated particle size was 125.845 nm, with an entrapment effectiveness of 74.669%. There was little difference between the actual and projected particle sizes and entrapment efficacy (
Figure 5).
It demonstrates that the model’s fit between them was appropriately modified.
Evaluation of TH_NLCopt gels
The TH_NLCopt gel was evaluated by measuring pH, spreadability, viscosity, homogeneity, extrudability, and drug content. The result observed from the evaluation test is mentioned in
Table 8.
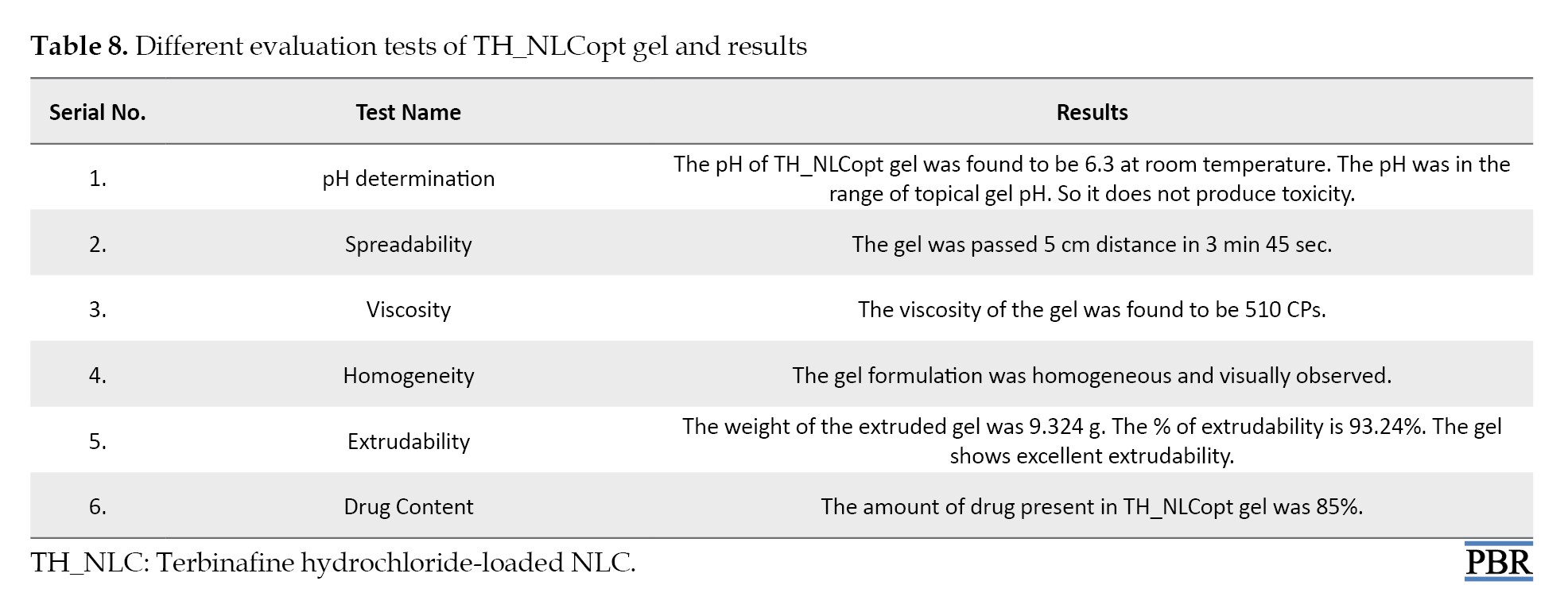
These results confirm the suitable physicochemical parameter of the TH_NLCoptgel for topical application.
In vitro drug release study using dialysis membrane
The in vitro drug release profiles of terbinafine hydrochloride from TH_NLCopt and TH_NLCopt gel are reported in
Figure 6.
The in vitro drug release study was performed for 7 hours. The results show that the drug release from the TH_NLCopt and TH_NLCopt gel formulations follows biphasic behavior. At first, a burst release of TH was observed, and after a certain time, the release of TH_NLCopt and TH_NLCopt gel slowed. This result predicted that the drug release from TH_NLCopt was greater than the TH_NLCopt gel. The release rate of terbinafine hydrochloride was 96.35% and 83.37% in 7 hours from TH_NLCopt and TH_NLCopt gel, respectively.
Ex vivo permeation study using goat abdominal skin
The ex vivo permeation profile of terbinafine hydrochloride from TH_NLCopt and TH_NLCopt gel has been plotted in
Figure 7.
The results show that the drug permeation from the NLC formulations gradually increased and maintained a constant rate after 6 hours. This result predicted that the drug permeated from TH_NLCopt and TH_NLCopt gel through goat abdominal skin was 54.19% and 31.16% in 7 hours, and the drug remained in the donor compartment at 23.35% and 6.97%, respectively. From there, the drug present in a different layer of the skin or drug retention was calculated for TH_NLCopt and TH_NLCopt gel, and the values were 22.46% and 61.87%, respectively. The permeation of Terbinafine Hydrochloride from TH_NLCopt and TH_NLCopt gel formulations through goat abdominal skin follows zero-order kinetics, and the R2 values were 0.9807 and 0.969, respectively. The permeation coefficient or permeability coefficient (P)=J/S×Cd, where S=effective surface area, J=slope of the line, Cd=drug concentration within the receiver chamber varies from 0.00602 cm/h to 0.00725 cm/h, and the flux was 0.0949 to 0.068 mg/cm2/min [
35].
Discussion
This study is carried out on TH_NLC, as mentioned above. The study was done to see the effectiveness of NLC for topical delivery of terbinafine hydrochloride.
At first, the compatibility between the drug and excipients was checked by conducting an FTIR study. By analyzing the FTIR spectra, it can be interpreted that stearic acid, olive oil, and the drug did not interact physically or chemically. One of the important pre-formulation studies to fabricate a new dosage form is drug–excipient compatibility. Among the various methods available to study it, FTIR-spectroscopy is an easy and simple way [
36]. FTIR spectroscopy shows interaction at the molecular level, which means the functional groups. In this work, the FTIR spectra (
Figure 1) suggest physical interactions due to the formation of weak to medium-intensity bonds since no major shifting of peaks was noted [
37]. Stretches found are mainly responsible functional groups for those regions. Few physical interactions might help sustain the release of drug molecules from the experimental formulations, which is a prerequisite for the current study.
Then, the formulation was designed with the help of Design-Expert® software using a two-factor and three-level face-centered central composite design to optimize the formulation based on particle size and entrapment efficiency as two responses. To check and interpret the error, 13 formulations were given by the software with 5 repetitions. The DLS study revealed that the particles were between 17.57 nm to 329.4 nm, proving nanostructure formation. The entrapment efficiency of the TH_NLC formulation ranged from 60.94% to 98%. An SEM image of the formulation confirmed the surface morphology and the particle size distribution of the TH_NLC1 formulation. The particle size was observed to be 52 nm to 176 nm by an SEM analysis of the TH_NLC1 formulation. The software interpreted the values of particle size and entrapment efficiency. The ANOVA was used to analyze the results. The effect of independent variables (factor) on the response parameter particle size and entrapment efficiency was described by polynomial equations and 3D response surface graphs produced by the software. The software performed point prediction, and the optimized formulation was selected. The optimized formulation (TH_NLCopt) has the following composition: 4 mL amount of liquid lipids and a homogenization speed of 3000 rpm. The composition displayed an entrapment efficiency of 75% and a particle size of 134 nm.
The optimized formulation was converted into the gel using carbopol as a gelling agent. The TH_NLCopt gel was evaluated by measuring pH, spreadability, viscosity, homogeneity, extrudability, and drug content. These results confirm the suitable physicochemical parameter of the TH_NLCopt gel for topical application.
The in vitro drug release study used a dialysis membrane, and the ex vivo permeation study used goat abdominal skin with TH_NLCopt and TH_NLCopt gel formulations using a Franz diffusion cell. The in vitro drug release study was performed for 7 hours. The results show that the drug diffusion from the TH_NLCopt and TH_NLCopt gel formulations follows biphasic behavior. At first, a burst release of TH was observed, and then after a certain time, it gets slow release from the TH_NLCopt and TH_NLCopt gel. This is due to the unentrapped drug diffusing quickly, and then the drug release gets slower. This result predicted that the drug release from TH_NLCopt was better than the TH_NLCopt gel. This outcome is due to the carbopol polymer in the gel, which resists the drug release from the gel. The biphasic release behavior is ideal for topical formulation because the burst release gives the amount of drug at the target site to achieve therapeutic concentration, and slow release helps maintain the therapeutic concentration.
This study aimed to target the drug with a controlled release effect on the skin. To achieve drug penetration into certain layers of the skin, NLCs and NLC-based gel as a supersaturated system have been used.
Percutaneous drug absorption is a process that includes the steps of dissolution and release of the drug from the vehicle/formulation, the partition of a drug into the stratum corneum, diffusion of the solubilized drug across the stratum corneum, and penetration of the drug into the layers of the skin [
38].
The ex vivo permeation study results show that the drug permeation from the TH_NLCopt and TH_NLCopt gel formulations gradually increased and maintained a constant rate after 6 hours. From this result, it was found that the permeation of terbinafine hydrochloride from TH_NLCopt was greater than that of TH_NLCopt gel. This outcome is due to the carbopol matrix. The slow permeation from TH_NLCopt gel is ideal for topical formulation. The drug slowly permeates the skin and retains on the skin for a longer period. The presence of the drug in the target area has a more prominent effect than the prepared NLC formulation. The results of the studies compared the drug diffusion profile and permeation profile of TH_NLCopt and TH_NLCopt gel formulations. Therefore, based on the outcome of the analysis performed here, we can state that the NLC-based gel formulations effectively affect the delivery of antifungal drugs through the skin (topical drug delivery).
Conclusion
This study developed and optimized the TH_NLC formulation to address two response parameter particle size and entrapment efficiency using a two-factor, three-level face-centered central composite design. The particle size of the NLCs was confirmed using the DLS technique and SEM. The indirect method was used to calculate the prepared TH_NLCs’ entrapment efficiency. Then, the optimized products selected by point prediction performed by the design Expert Software were incorporated into the hydrogel and evaluated by measuring pH, spreadability, viscosity, homogeneity, extrudability, and drug content. These results confirm the suitable physicochemical parameter of the TH_NLCopt gel for topical application. The in vitro drug release study using a dialysis membrane and the ex vivo permeability study were performed to compare the TH_NLCopt with TH_NLCopt gel, which is better for topical formulation. This study stated that the terbinafine hydrochloride-loaded nanostructured lipid carrier-based hydrogel system is potentially active for topical antifungal drug delivery.
We used ANOVA as a statistical method to detect any non-significant components in the study. The ANOVA result was deemed significant since the F value showed a value >4. Residual values were found to support the optimization, and the degree of lack of fit was deemed negligible. The model seemed significant because the P<0.05, precisely 0.0335 for particle size and <0.0001 for entrapment efficiency. A calculation of the software’s accuracy as a percentage prediction was done. The interaction between independent and dependent variables is encouraged by the polynomial equation’s positive sign, whereas the reverse relationship is shown by its negative value.
Ethical Considerations
Compliance with ethical guidelines
There were no ethical considerations to be considered in this research.
Funding
This research did not receive any grant from funding agencies in the public, commercial, or non-profit sectors.
Authors' contributions
Conceptualization and supervision: Gopa Roy Biswas; Methodology: Soumik Patra and Pritam Dutta; Investigation and writing the original draft: Gopa Roy Biswas and Pritam Dutta; Data collection and analysis: Gopa Roy Biswas, Soumik Patra, and Ritu Khanra; Review, editing and final approval: All authors.
Conflict of interest
The authors declared no conflict of interest.
Acknowledgments
The authors thank the institutions for providing the necessary research facilities. They are also thankful to Bose Institute, Kolkata, for providing the experimental support of DLS and CRNN, and the University of Calcutta, for providing the experimental support of SEM.
References
- Yadav RP, Chauhan MK. Terbinafine loaded chitosan scaffold for fungal wound treatment. Int J Pharm Sci Res. 2020; 11(8):3806-18. [DOI:10.13040/IJPSR.0975-8232.11(8).3806-18]
- Thavva V, Baratam SR. Formulation and evaluation of terbinafine hydrochloride microsponge gel. Int J Appl Pharm. 2019; 11(6). 78-85. [DOI:10.22159/ijap.2019v11i6.32502 ]
- Jain PS, Chaudhari AJ, Patel SA, Patel ZN, Patel DT. Development and validation of the UV-spectrophotometric method for determination of terbinafine hydrochloride in bulk and in formulation. Pharm Methods. 2011; 2(3):198-202. [DOI:10.4103/2229-4708.90364] [PMID]
- Arpa MD, Ünükür MZ, Erim ÜC. Formulation, characterization and in vitro release studies of terbinafine hydrochloride loaded buccal films. J Res Pharm. 2021; 25(5):667-80 [DOI:10.29228/jrp.58 ]
- Paliwal S, Kaur G, Arya RA. Formulation and characterization of topical nano emulgel of terbinafine. Univers J Pharm Res. 2019; 3(6). [DOI:10.22270/ujpr.v3i6.223]
- Gaba B, Fazil M, Khan S, Ali A, Baboota S, Ali J. Nanostructured lipid carrier system for topical delivery of terbinafine hydrochloride. Bull Fac Pharm Cairo Univ. 2015; 53(2):147-59. [DOI:10.1016/j.bfopcu.2015.10.001 ]
- Kaur S, Nautyal U, Singh R, Singh S, Devi A. Nanostructure Lipid Carrier (NLC): The new generation of lipid nanoparticles. Asian Pac J Health Sci. 2015; 2(2):76-93. [DOI:10.21276/apjhs.2015.2.2.14 ]
- Sharma A, Baldi A. Nanostructured lipid carriers : A review. J Dev Drugs. 2018; 7(1):1-13. [Link]
- Haider M, Abdin SM, Kamal L, Orive G. Nanostructured lipid carriers for delivery of chemotherapeutics: A review. Pharmaceutics. 2020; 12(3):288. [DOI:10.3390/pharmaceutics12030288 ] [PMID]
- Ameeduzzafar, Qumber M, Alruwaili NK, Bukhari SNA, Alharbi KS, Imam SS, et al. BBD-Based Development of Itraconazole Loaded Nanostructured Lipid Carrier for Topical Delivery: In Vitro Evaluation and Antimicrobial Assessment. J Pharm Innov. 2021; 16(1):85-98. [DOI:10.1007/s12247-019-09420-5 ]
- Baghel S, Nair VS, Pirani A, Sravani AB, Bhemisetty B, Ananthamurthy K, et al. Luliconazole-loaded nanostructured lipid carriers for topical treatment of superficial Tinea infections. Dermatol Ther. 2020; 33(6):e13959. [DOI:10.1111/dth.13959 ]
- Syed Azhar SNA, Ashari SE, Zainuddin N, Hassan M. Nanostructured lipid carriers-hydrogels system for drug delivery: Nanohybrid technology perspective. Molecules. 2022; 27(1):289. [DOI:10.3390/molecules27010289 ] [PMID]
- Sethuraman N, Shanmuganathan S, Sandhya K, Anbarasan B. Design, development and characterization of nano structured lipid carrier for topical delivery of aceclofenac. Indian J Pharm Educ Res. 2018; 52(4):581-6. [Link]
- Tran TH, Ramasamy T, Truong DH, Choi HG, Yong CS, Kim JO. Preparation and characterization of fenofibrate-loaded nanostructured lipid carriers for oral bioavailability enhancement. AAPS PharmSciTech. 2014; 15(6):1509-15.[DOI:10.1208/s12249-014-0175-y ] [PMID]
- Jain K, Sood S, Gowthamarajan K. Optimization of artemether-loaded NLC for intranasal delivery using central composite design. Drug Deliv. 2015; 22(7):940-54. [DOI:10.3109/10717544.2014.885999 ] [PMID]
- Hassan H, Adam SK, Alias E, Meor Mohd Affandi MMR, Shamsuddin AF, Basir R. Central composite design for formulation and optimization of solid lipid nanoparticles to enhance oral bioavailability of acyclovir. Molecules. 2021; 26(18):5432. [DOI:10.3390/molecules26185432 ] [PMID]
- Riaz A, Hendricks S, Elbrink K, Guy C, Maes L, Ahmed N, et al. Preparation and characterization of nanostructured lipid carriers for improved topical drug delivery: Evaluation in cutaneous leishmaniasis and vaginal candidiasis animal models. AAPS PharmSciTech. 2020; 21(5):185. [DOI:10.1208/s12249-020-01717-w] [PMID]
- Geng Q, Zhao Y, Wang L, Xu L, Chen X, Han J. Development and evaluation of astaxanthin as nanostructure lipid carriers in topical delivery. AAPS PharmSciTech. 2020; 21(8):318. [DOI:10.1208/s12249-020-01822-w ] [PMID]
- Patil D, Pattewar S, Palival S, Patil G, Sharma S. Nanostructured lipid carriers: A platform to lipophilic drug for oral bioavailability enhancement. J Drug Deliv Ther. 2019; 9(3):758-64.[DOI:10.22270/jddt.v9i3-s.2750]
- Rao S, Barot T, Rajesh KS, Jha LL. Formulation, optimization and evaluation of microemulsion based gel of Butenafine Hydrochloride for topical delivery by using simplex lattice mixture design. J Pharm Investig. 2016; 46:1-12. [DOI:10.1007/s40005-015-0207-y]
- Iizhar SA, Syed IA, Satar R, Ansari SA. In vitro assessment of pharmaceutical potential of ethosomes entrapped with terbinafine hydrochloride. J Adv Res. 2016; 7(3):453-61. [DOI:10.1016/j.jare.2016.03.003 ] [PMID]
- Badawi NM, Elkafrawy MA, Yehia RM, Attia DA. Clinical comparative study of optimized metronidazole loaded lipid nanocarrier vaginal emulgel for management of bacterial vaginosis and its recurrence. Drug Deliv. 2021; 28(1):814-25. [DOI:10.1080/10717544.2021.1912211] [PMID]
- Alam MS, Algahtani MS, Ahmad J, Kohli K, Shafiq-Un-Nabi S, Warsi MH, et al. Formulation design and evaluation of aceclofenac nanogel for topical application. Ther Deliv. 2020; 11(12):767-78. [DOI:10.4155/tde-2020-0076 ] [PMID]
- Shimojo AAM, Fernandes ARV, Ferreira NRE, Sanchez-Lopez E, Santana MHA, Souto EB. Evaluation of the influence of process parameters on the properties of resveratrol-loaded NLC using 22 full factorial design. Antioxidants. 2019; 8(8):272. [DOI:10.3390/antiox8080272 ] [PMID]
- Varela-Fernández R, García-Otero X, Díaz-Tomé V, Regueiro U, López-López M, González-Barcia M, et al. Lactoferrin-loaded nanostructured lipid carriers (NLCs) as a new formulation for optimized ocular drug delivery. Eur J Pharm Biopharm. 2022; 172:144-56. [DOI:10.1016/j.ejpb.2022.02.010 ] [PMID]
- Patil TS, Gujarathi NA, Aher AA, Pachpande HE, Sharma C, Ojha S, et al. Recent advancements in topical anti-psoriatic nanostructured lipid carrier-based drug delivery. Int J Mol Sci. 2023; 24(3):2978. [DOI:10.3390/ijms24032978 ] [PMID]
- Khurana S, Jain NK, Bedi PM. Development and characterization of a novel controlled release drug delivery system based on nanostructured lipid carriers gel for meloxicam. Life Sci. 2013; 93(21):763-72. [DOI:10.1016/j.lfs.2013.09.027 ] [PMID]
- Teng Z, Yu M, Ding Y, Zhang H, Shen Y, Jiang M, et al. Preparation and characterization of nimodipine-loaded nanostructured lipid systems for enhanced solubility and bioavailability. Int J Nanomedicine. 2018; 14:119-33. [DOI:10.2147/IJN.S186899 ] [PMID]
- Doktorovova S, Souto EB. Nanostructured lipid carrier-based hydrogel formulations for drug delivery: A comprehensive review. Expert Opin Drug Deliv. 2009; 6(2):165-76. [DOI:10.1517/17425240802712590 ] [PMID]
- Puri V, Froelich A, Shah P, Pringle S, Chen K, Michniak-Kohn B. Quality by design guided development of polymeric nanospheres of terbinafine hydrochloride for topical treatment of onychomycosis using a nano-gel formulation. Pharmaceutics. 2022; 14(10):2170. [DOI:10.3390/pharmaceutics14102170 ] [PMID]
- Mahmood A, Rapalli VK, Gorantla S, Waghule T, Singhvi G. Dermatokinetic assessment of luliconazole-loaded nanostructured lipid carriers (NLCs) for topical delivery: QbD-driven design, optimization, and in vitro and ex vivo evaluations. Drug Deliv Transl Res. 2022; 12(5):1118-35. [DOI:10.1007/s13346-021-00986-7 ] [PMID]
- Biswas GR, Chakraborty S, Ghosh N, Majee SB. Fabrication of bucco-matrix tablets of Amoxicillin trihydrate on the basis of release and permeation kinetics. J App Pharm Sci. 2015; 5(04):048-52. [DOI:10.7324/JAPS.2015.50408]
- Szabó P, Daróczi TB, Tóth G, Zelkó R. In vitro and in silico investigation of electrospun terbinafine hydrochloride-loaded buccal nanofibrous sheets. J Pharm Biomed Anal. 2016; 131:156-9. [DOI:10.1016/j.jpba.2016.08.021 ] [PMID]
- Gul U, Khan MI, Madni A, Sohail MF, Rehman M, Rasul A, et al. Olive oil and clove oil-based nanoemulsion for topical delivery of terbinafine hydrochloride: In vitro and ex vivo evaluation. Drug Deliv. 2022; 29(1):600-12. [DOI:10.1080/10717544.2022.2039805 ] [PMID]
- Biswas GR, Majee SB, Roy A. Combination of synthetic and natural polymers in hydrogel: An impact on drug permeation. J Appl Pharm Sci. 2016; 6(11):158-64. [DOI:10.7324/JAPS.2016.601125 ]
- Brittain HG. Physical characterizations of pharmaceutical solids. Boca Raton: CRC Press; 1995. [DOI:10.1201/9780367802967 ]
- Kötting C, Gerwert K. Monitoring protein-ligand interactions by time- resolved FTIR difference spectroscopy. Methods in Molecular Biology. Totowa: Humana; 2005. [DOI:10.1385/1-59259-912-5:261 ]
- Muller RH, Wissing S. SLN and lipopearls for topical delivery of active compounds and controlled release. Drugs Pharm Sci. 2003; 126:571-88. [DOI:10.1201/9780203910337.ch47 ]