Rahamouz-Haghighi S. Biological Activities and Analytical Methods for Detecting Aucubin and Catalpol Iridoid Glycosides in Plantago Species: A Review Study. Pharm Biomed Res 2023; 9 (2) :85-114
URL:
http://pbr.mazums.ac.ir/article-1-493-en.html
Department of Plant Production and Genetics, Faculty of Agriculture, University of Zanjan, Zanjan, Iran.
Full-Text [PDF 3604 kb]
(1432 Downloads)
|
Abstract (HTML) (1988 Views)
Full-Text: (2543 Views)
Introduction
Iridoid was first isolated at the end of the 19th century. But, the principal structure of the iridoid was identified in 1958 by Halpern and Schmid. Then, several scientific studies were conducted on iridoids relating to agriculture, biosynthesis, botany, and medicinal uses. Up to now, hundreds of iridoids have been recognized in diverse sources [1]. Iridoids are categorized into iridoid glycosides, non-glycosidic iridoids or aglycone, bisiridoids, and secoiridoids groups [2]. Iridoid glycosides are monoterpenes in at least 57 plant families [3]. Their function in plants is mostly protection [4]. Iridoids can be found in the following families: Lamiaceae, Acanthaceae, Plantaginaceae, Gentianales, Cornales Scrophulariaceae, and Rubiaceae [5, 6, 7, 8]. Especially, aucubin and catalpol are found in plant subclasses of Asteridae, including Loganiaceae, Lamiaceae, Ericaceae, Gentianaceae, Verbenaceae, Rubiaceae, Oleaceae, Scrophulariaceae, Valerianaceae, Plantaginaceae, and Menyanthaceae [9].
Consequently, studies on Plantago species have just established growing attention owing to valuable components in these plants, such as aucubin and catalpol. So, the present study reviewed the cytotoxic properties and detection methods of aucubin and catalpol to create a comprehensive reference for utilizing these compounds.
Aucubin and Catalpol Names
Aucubin is known with CAS: 479-98-1 and chemical formula: (2S,3R,4S,5S,6R)-2-[[(1S,4aR,5S,7aS-5-hydroxy-7-(hydroxymethyl)-1,4a,5,7a-tetrahydrocyclopenta[c]pyran-1-yl]oxy]-6-(hydroxymethyl)oxane-3,4,5-triol [10].
Compound summary
Catalpol is introduced with CAS: 2415-24-9 and chemical formula: (2S,3R,4S,5S,6R)-2-{[(1aS,1bS,2S,5aR,6S,6aS)-6-Hydroxy-1a-(hydroxymethyl)-1a,1b,2,5a,6,6ahexahydrooxireno[20,30:4,5]cyclopenta[1,2-c]pyran-2-yl]oxy}-6-(hydroxymethyl)oxane-3,4,5-triol [10].
Aucubin and catalpol are iridoid glycosides used in herbal medicine (Figure 1).
Biosynthesis of Aucubin and Catalpol
Iridoids are a group of cyclopentanone monoterpenes found in plants as glycosides and regularly linked to glucose at C-1. The backbone of carbocyclic iridoids (Figure 2) is generally a cyclopentane unit attached to a dihydropyran ring.
On the other hand, secoiridoids are formed due to C-7/C-8 cleavage. These compounds have been classified as chemotaxonomic markers, and their presence provides evidence to explain many species whose taxonomic boundaries are unclear [11, 12].
Iridoids are often found as glucosides, featuring a b-D-glucopyranosyl unit attached at C-l via a b-hemiacetalic bond (R = glucose) [13].
There are two main biosynthetic pathways for producing iridoids (Figure 3).
The first pathway makes compounds that generally originate in Gentianales and Cornales orders. In this pathway, deoxyloganic acid is synthesized, the precursor of many iridoids with 8β stereochemistry, such as secologanin and loganin. The latter is caused by the oxidative cleavage of the C-7/C-8 linkage of the cyclopentane ring. After an intricate synthesis including tryptamine, secologanin gives rise to indole alkaloids; vinblastine, vincristine, and reserpine, among others, are regularly discovered in the families Rubiaceae, Apocynaceae, and Loganiaceae, order Gentianales. Another group of iridoids is biosynthetically created by the second pathway. This pathway produces 8-epi-deoxyloganic acid, a precursor to iridoids with 8-a carbon substituent, and both C-4 carboxylated and C-4 decarboxylated carbocyclic iridoids, for example, ipolamiide and aucubin, respectively. These compounds are almost particularly established in families of Lamiales [14].
Rønsted et al. have revealed the biosynthesis of aucubin and catalpol, mainly in Plantago major (Figure 4) [15].
Distribution of Aucubin and Catalpol in the Plant Kingdom
Aucubin was first identified in Aucuba japonica in 1905. However, it is also found in many other natural plants such as Plantago asiatica L, Eucommia ulmoides Oliv., and Aucuba japonica Thunb [16]. Aucubin is the most common compound in the iridoid glycoside class [8]. Aucubin is also an intermediate of catalpol (Figure 3) [17]. Catalpol iridoid glucoside is broadly dispensed in many plant families and is mainly acquired from Rehmannia glutinosa Libosch root [18]. The selection for catalpol may have resulted in a reduction in aucubin concentration [19]. Aucubin and catalpol can be applied as potential chemotaxonomic markers to regulate the quality of different plant extracts, such as Plantago species [20].
Plantain is known to have two important aucubin and catalpol compounds [21]. Aucubin is present in almost all Plantago species, whereas catalpol or its derivatives have been reported in some species [22]. Iridoid glycoside concentration also changes with the plant’s developmental parts, age, genetic, and environmental factors such as weather, time of day, soil status, and arbuscular mycorrhizal fungi [3, 23-35]. Temperature, UV light, and soil nutrient conditions can alter the content of the secondary metabolites of plantain [36]. In some studies, the mean content of catalpol in the leaves of P. lanceolata was lower than the aucubin content [33, 37, 38 ], although, in one study, the opposite state was reported [3]. The contents of catalpol in P. lanceolata interrelated adversely with leaf age and the total number of leaves [35]. In Bowers and Stamp’s study, in genotypes of P. lanceolata, the amount of aucubin was more in intermediate leaves compared to mature leaves, while catalpol content was higher in intermediate and young leaves [25]. Increasing leaf age leads to an increase in aucubin content relative to total iridoid glycosides [25]. On the other hand, genotype significantly affects iridoid glycoside content, although an individual plant is quite heterogeneous in terms of iridoid glycoside content. New leaves have twice as many iridoid glycosides as mature leaves [25]. Lastly, Bowers and Stamp concluded that as leaves age, less catalpol is produced, breaks down faster, and translocation occurs in the leaf [25]. Lampert and Bowers indicated that aucubin is most in old leaves, while catalpol is high in young ones [39]. The amounts of aucubin and catalpol and the ratio of catalpol to total iridoid glycosides are impassioned by interactions between the time of harvest and leaf age [40]. The amounts of catalpol in new leaves increase between the harvests, although, in intermediate and mature leaves, harvest date showed no significant influence on catalpol content [40].
De Deyn et al. showed that among the full sibling families of P. lanceolata, plants contained high constitutive levels of defensive iridoid glycosides in leaves and roots. They reported that the concentration of aucubin was higher than that of catalpol and was found more in the root than in the shoot tissue [41]. The difference in constitutive iridoid glycoside values in P. lanceolata is genetically regulated to some extent [25, 26, 27, 32].
Furthermore, environmental and ontogeny strongly affect iridoid glycoside amounts in P. lanceolata. Seasonal changes probably affect the content of bioactive compounds in plantain leaves in different ways. Solar emission, nutrient disposal, and air temperature are major issues. Average levels differ among habitats and populations [36] and are mostly higher in plants grown under high light and low nutrient or water situations [28, 37]. Tamura reported that nitrogen source and low light intensity forcefully suppressed the accumulation of aucubin in plantain leaves but had no effect on catalpol concentration [36]. Tamura also explained that relatively high air temperatures (20°C/18°C, day/night) enhanced aucubin and catalpol contents. Therefore, plants grown under high temperatures can accumulate higher aucubin than those produced in low air temperatures (15°C/10°C, day/night) [36]. In total, Tamura and Nishibe assumed that the content of aucubin is more when the air temperature is optimal for growth [34].
Tamura and Nishibe investigated the effect of seasonal changes in the content of bioactive compounds in plantain leaves [34]. The amount of aucubin increased from late spring to midfall, but in midsummer, aucubin levels were relatively constant. In late fall, aucubin levels gradually decreased in Grasslands Lancelot (0.13% °C-1) and Ceres Tonic (0.20% °C-1), relative to the decline in air temperature from 14.7°C to 10.7°C. However, in midfall, when the temperature was still around 20°C, the levels of aucubin in both cultivars’ leaves were the highest. On other hand, catalpol content was very low relative to the contents of aucubin. The seasonal changes in the amount of catalpol were less visible than in aucubin, so the concentration of catalpol increased slightly during the growing season, but its amount was low in the middle of summer. The less clear-cut changes in catalpol can be due to the low base level of its synthesis, which does not respond to environmental changes. However, in Nieminen et al. study, catalpol contents were more than aucubin in mid- and late-summer [42].
Bowers et al. also studied seasonal variations in aucubin and catalpol amounts in plantain leaves [43]. Plants were harvested at 2-week intervals from late spring to early fall (4 times). They showed a significant increase in aucubin and catalpol content during the growing season, but the levels of the compounds decreased in plants harvested in mid-summer compared to those harvested on the other three sampling dates. Since the air temperature in midsummer was very high (around 25-30°C), this result agrees with the previous explanation [34]. Furthermore, P. lanceolata stores more catalpol when grown in soils conditioned by grass species [44].
It is usually presumed that medicinal plants, for their pharmacological uses, should be dried at less than 60°C to minimize the loss of bioactive compounds. Therefore, the concentration of bioactive compounds gradually degenerated in the early drying stages. The reason for the decay in the amounts of catalpol and aucubin could be the presence of enzymes in charge of their degradation. The degradation of iridoid glycosides is because of β-glucosidase action. The activities of these putative enzymes can be neutralized after about 3 and 24 h after the start of drying under natural climatic status and at 60°C, respectively since the compounds’ levels are fixed after these times [34]. Finally, it is suggested that midfall is the best time to harvest plantain for medicinal purposes because the amounts of the active compounds progressively decrease in the early stages of drying both under natural climatic conditions and at a temperature of 60°C [34].
Aucubin and Catalpol Content in Different Plant Parts and In Vitro Cultures
Plantain is applied in traditional medicines and for pasture. As that aucubin is a precursor in catalpol biosynthesis, several studies assessed the content of iridoid glycosides in plantain under different conditions, including the following studies. Aucubin is formed in plantain at very high levels, up to 3% of dry weight, depending on various aspects of the genotype, soil fertility, and so on [28, 32, 45]. The content of iridoid glycosides, including aucubin and catalpol, in a natural population of P. lanceolata, can attain even 9% of dry matter [3]. With increasing leaf age and dry summer conditions, the levels of these compounds increase. Also, cutting the surfaces in detached leaves can significantly increase them [25, 30, 46].
Another study reported that the level of aucubin and catalpol in seven Plantago species was up to 0.27% and 1.81% of dry leaf weight, respectively [47]. Furthermore, aucubin and catalpol production increased over time from 0.003% to 8.86% dry weight from seeing seedling pre-reproductive plants [48]. A significant interaction between plant age and tissue indicated differences in iridoid glycoside content between shoots and roots during plant growth. The results are presented in three cases. First, the average of iridoid glycosides was three times higher in the shoots compared to the roots. Second, the mean group differences in aucubin and catalpol among all 7 age classes were similar for the shoot and root tissues. Third, the concentration of aucubin in the shoot compared to the total iridoid glycosides caused more changes during plant ontogeny [48]. Pellissier et al. noted that total iridoid glycosides content, particularly for catalpol, was low (1%–7%) compared to Bowers et al., Stamp and Bowers, and Marak et al. studies [43, 46, 49, 50]. Remarkably, in the two works in which laboratory-reared plants were applied, catalpol contents were exceptionally low (0%–0.6%) [37, 38]. Consequently, Iridoid glycosides contents were lower in the greenhouse-grown plants (0.83±0.09 to 6.41±1.02 mg/g) than in field-grown plants (1.47±0.26% to 8.63±0.26%) [51]. The aucubin content in plants harvested from the field was more than 1.6%–2.7% in Bowers, 0.5%–5% in Darrow and Bowers, and 0.6%–2.2% in Nieminen et al. [3, 33, 42]. Catalpol concentrations were comparable in these studies 0.4%–3.6% in Bowers [3], 0.2%–2.2% in Darrow and Bowers [33], and 0.7%–2.0% in Nieminen et al. [42].
Generally, the aucubin amounts of leaves increased throughout the growing season, ranging from 0.5% to 4%-5% dry matter from July to September and October, respectively. Likewise, the catalpol content of leaves was calculated to be lower in July and increase throughout the season until October. However, it revealed that the catalpol content in the leaves was about half that of aucubin, ranging from about 0.25% dry matter to about 2.5% dry matter. In the reproductive stalks, the aucubin and catalpol levels tended to increase gradually during the early part of the season and decrease sharply between September and October. The total iridoid glycoside content in the reproductive stalks was slightly lower than in the leaves: 1%-5% and 1%-7%, respectively. While the leaves had higher levels of aucubin than catalpol, the reproductive tissues had more contents of catalpol than aucubin [33].
In another investigation, two cultivars of P. lanceolata L., i.e., Ceres Tonic and Grasslands Lancelot, were seeded in spring. The difference in aucubin and catalpol levels in the leaves during the growing season and by drying post-harvesting were quantitatively evaluated using high-performance liquid chromatography (HPLC). The content of catalpol was relatively low (between 1% and 2% of dry matter) throughout the growing season, and there was no obvious seasonal change. From spring to midfall, the aucubin content gradually increased from 1.0% to 2.7% in Ceres Tonic and from 2.1% to 4.8% in Grasslands Lancelot [34].
Navarrete et al. reported the level of catalpol and aucubin in plantain (cv. Ceres Tonic) during two successive growing seasons (2011–2012 and 2012–2013) [52]. There was almost no concentration of catalpol in plantain (cv. Ceres Tonic), but aucubin concentrations increased during the growing season. The content of aucubin increased from 1.78 to 3.80 mg/g dry matter in the first and from 0.44 to 6.87 mg/g dry matter in the second growing season. In late fall, aucubin amounts steadily decreased in Grasslands Lancelot and Ceres Tonic. In this regard, the content of aucubin and catalpol in different parts of Plantago species has been assessed by researchers with various methods (Table 1).



Aucubin and Catalpol Preparation for Analysis
Aucubin is soluble in water. However, it spontaneously undergoes oxidation and forms insoluble components in aqueous solutions. It is also soluble in methanol and ethanol but is insoluble in organic solvents, such as benzene, chloroform, ether, and petroleum ether [72]. Iridoid glycosides usually tend to be hydrolyzed and undergo rearrangement under slightly acidic conditions [73]. Therefore, they must be treated and analyzed under strictly alkaline conditions. However, some structures are more unstable and may hydrolyze even under alkaline conditions or upon heating [74]. As aucubin is extracted from plants, it is suggested that aucubin be prepared at a low temperature in a weak acidic condition and dark status to increase its output and stability [75]. In Nieminen et al. study, hot water extraction was applied to separate the compounds, which was reproducible and easy. As well, it avoided the application of organic solvents, which burden the environment [42].
As a result, the dry methanolic extract was dissolved in water and partitioned with ETAC (ethyl acetate); for aucubin, only the aqueous layer gave a positive result in the Trim-Hill test [70]. The test (blue color) indicates the presence of iridoid glycosides.
Various methods for the extraction of aucubin have been developed according to its different chemical and physical properties, including cold maceration and reflux extraction. Special enzymes and ultrasound techniques to destroy the plant cell wall have been recommended to increase the permeability of active substances through the cell wall. Extraction by ultrasonic and enzymolysis help isolate aucubin. Also, the microwave extraction method has been used to extract aucubin from Eucommia ulmoides. Li et al. investigated the efficiency of the supercritical CO2 and Soxhlet extraction methods for aucubin from Eucommia ulmoides Oliv seeds. The findings showed that supercritical CO2 extraction provides a higher yield and lower extraction cost [75].
Analytical Methods and Techniques for Determination of Aucubin and Catalpol
Bioactive components are determined by expensive analytical methods that require chemicals, time, high competence, and know-how [76].
Commonly, iridoid glycosides (IG) are detected with chromatographic techniques, i.e., gas chromatography (GC), liquid chromatography (LC), or thin-layer chromatography (TLC) [42]. The IG contents (aucubin and catalpol) were analyzed by GC using previously described methods [26, 31, 37, 40, 77, 78, 79]. Some of the studies performed by GC analysis are listed in Table 2.

Flash chromatography was applied to separate catalpol from aucubin [83]. However, the TLC method was the first option for isolating aucubin and catalpol from the plant extracts [84-86]. Taskova et al. [87] used TLC to investigate iridoids from 44 Bulgarian collections selected from 14 species of Plantago, of which 14 compounds were determined using spectral methods [47]. The aqueous fraction was found to be rich in iridoids by the TLC technique [88]. In this regard, several studies applied analytical methods of high-performance thin-layer chromatography (HPTLC) and TLC for detecting aucubin and catalpol, summarized in Table 3.

Derivatization with other compounds is necessary for the visualization of the spot. So, reagents were used for post-derivatization of aucubin and catalpol, such as 10% alcoholic H2SO4, which burns the glucose molecule in the aucubin giving colored spots, and 10% anisaldehyde which gives a colored spot for aucubin. These reagents are applied by dipping TLC plates in the reagent solutions or by spraying the solutions on the TLC plates [70]. An ethanolic acidic solution of vanillin (1 mL H2SO4 conc., 3 g vanillin, 100 mL EtOH) is used to visualize iridoid glycosides as colored spots.
Rønsted et al. identified the isolated compounds by nuclear magnetic resonance spectroscopy [15, 22]. Their findings showed that the distribution pattern of the iridoids in 34 species of Plantago has a good correlation with the classification of Rahn [89]. The qualitative studies of Plantago atrata, Plantago bellardii, Plantago coronopus, Plantago holosteum, and Plantago reniformis have been reported [15, 87]. In some studies, aucubin and catalpol have been isolated from the genus Plantago (Table 4).
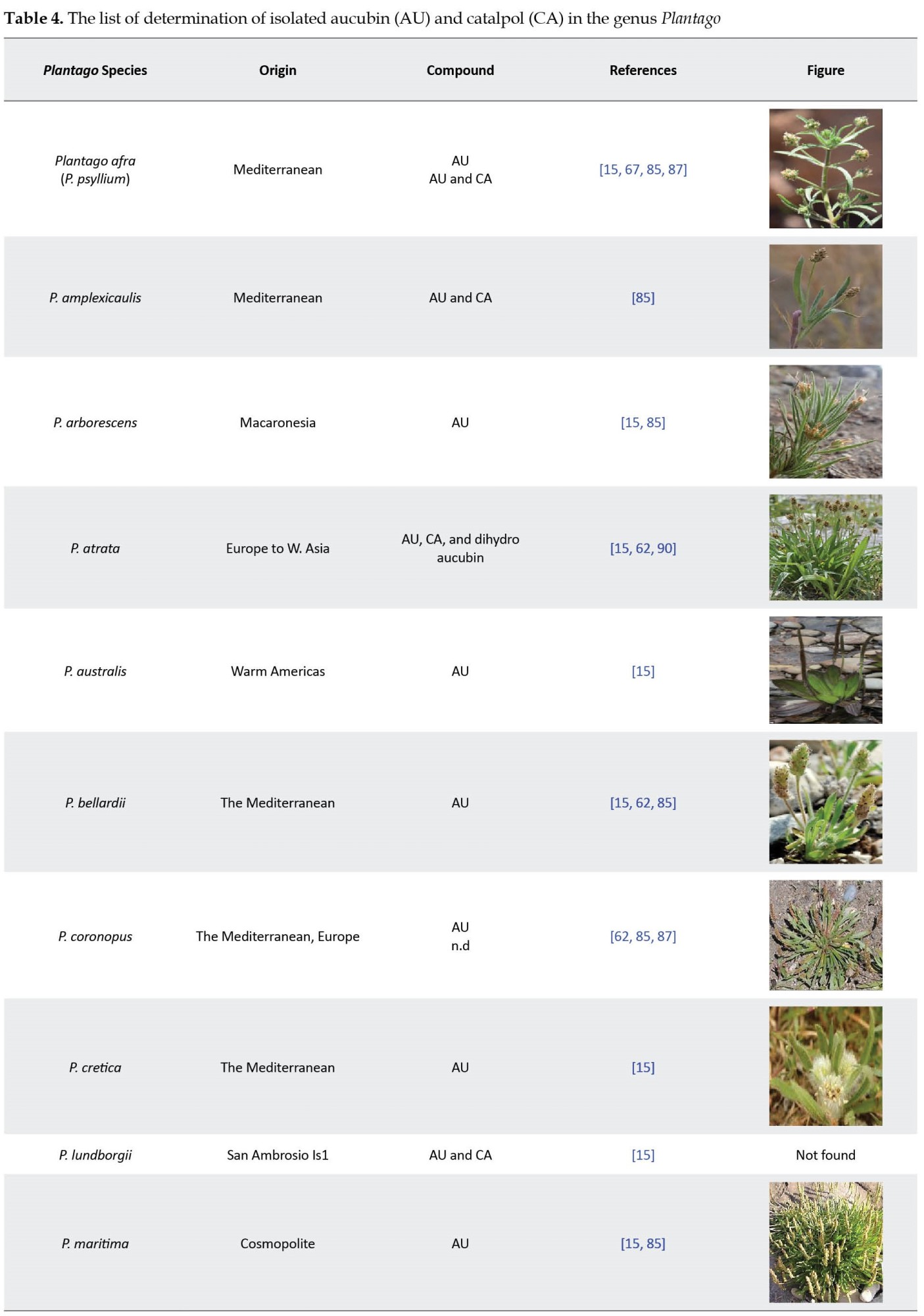



To quality control herbal samples containing iridoids, it is possible to quantify the levels of various iridoids in a mixed status by a simple method using liquid chromatography equipped with an ultraviolet detector (LC-UV) [60]. Usually, relatively large amounts of weak acids, such as phosphoric acid and acetic acid, are added to the mobile phase to prevent interference and stabilization of tautomeric rearrangements. In this way, a good separation of different iridoids can be achieved [114]. Kim et al. developed a simple LC-UV procedure to overcome such unfavorable interference by adding a small amount of trifluoroacetic acid (TFA) to the mobile phase with aqueous acetonitrile. They could simultaneously determine a small mixture of catalpol and aucubin in the aqueous extract of leaves, seeds, and roasted seeds of P. asiatica [60]. Aucubin and catalpol in the P. lanceolata extract were evaluated using liquid chromatography-mass spectrometry (LC-MS) [68, 70]. HPLC and HPTLC are frequently used to identify bioactive in samples in variety selection programs or to control new varieties [59, 115]. However, several methods can be applied to detect aucubin levels in Plantago extracts; the most common ones are HPLC [34, 116] and HPTLC [117, 118] methods. According to this, an attempt was also made for the quantitative analysis of aucubin in 7 Plantago species using HPLC and HPTLC by Janković et al. [62]. Other researchers also determined these compounds in Plantago species (Table 5).

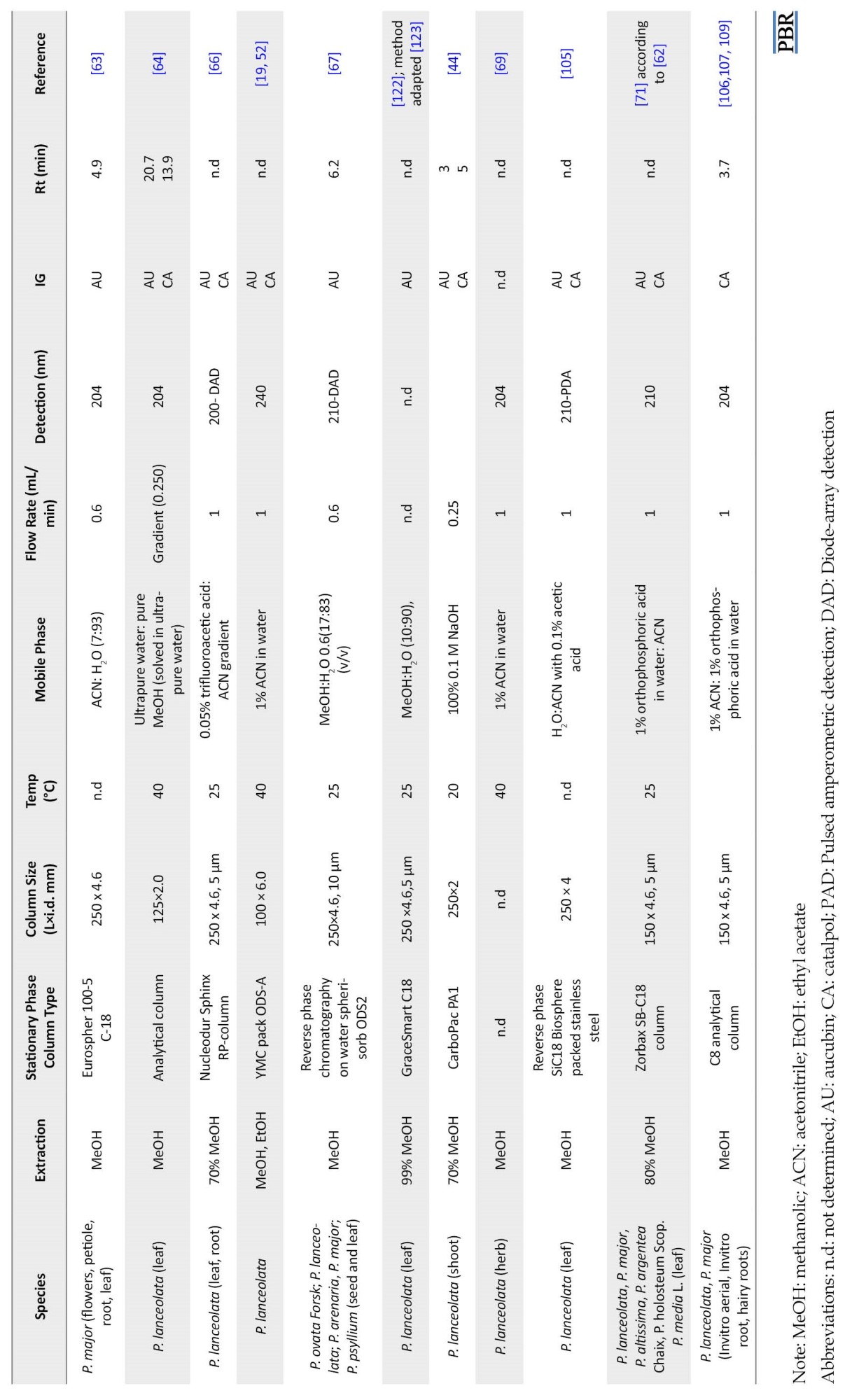
One of the conditions of HPLC is the absorption spectrum. The absorption spectra of aucubin (220, 255, 290 nm) are used for detecting aucubin extracted from plants [124].
Capillary electrophoresis (CE) is a valuable tool for medicinal plant quality handling, screening, and analysis [65]. All main bioactive secondary metabolite groups can be assayed by one of the CE techniques; MEKC (capillary zone electrophoresis [CZE], Micellar electrokinetic chromatography [MEKC], microemulsion electrokinetic chromatography [MEEKC], and nonaqueous CE [NACE]) [125]. The analysis of iridoids by the CE method has already been published [2, 126, 127]. CE methods detect metabolites such as iridoid glycosides in Plantago species [2].
The CE and micellar electrokinetic capillary chromatography (MECC) methods were also used for evaluating neutral compounds like aucubin and catalpol [47, 128]. CE method has been confirmed to be suitable for the quantitative determination of aucubin and catalpol from aqueous extracts of leaf parts of P. lanceolata, P. major, P. asiatica, and P. lanceolata callus [65]. The content of aucubin and catalpol in P. lanceolata was reliably and quickly determined by MECC [42].
Micellar electrokinetic chromatography (MEKC) was used to isolate and analyze aucubin and catalpol in hot water extraction of several Plantago species growing in Croatia: P. altissima L., P. argentea Chaix, P. coronopus L., P. holosteum Scop. (subsp. depauperata, subsp. holosteum and subsp. scopulorum), P. lagopus L., P. lanceolata L., and P. maritima L. Significant differences were exhibited between the iridoid contents in mentioned species using this method [47].
An authentic and simple CE-MEKC method has been validated and developed to quantitatively determine aucubin and catalpol of Plantago species, P. lanceolata calli, P. lanceolata matrices, P. altissima, P. major, P. media, and P. maritima [61]. However, TLC pattern analysis could recognize the species mentioned above in a single run in a system commonly applied for the quality management of P. lanceolata leaves. However, P. altissima and P. lanceolata did not represent enough pattern difference to be fully isolated [61]. Consequently, according to iridoid content, P. altissima was chemically indistinguishable from P. lanceolata [61].
Also, Gonda et al. evaluated the changes in aucubin and catalpol concentration in dry leaves of P. lanceolata subjected to the atmosphere with different relative humidity (0%, 45%, and 75%) by CE‐MEKC for 24 weeks [115]. CE-MEKC method showed that it is suitable for aqueous extracts of P. lanceolata, P. major, P. asiatica leaves, and P. lanceolata callus culture [65].
In another study, aucubin from P. lanceolata was separated and quantified by preparative TLC and then determined by HPTLC fingerprinting. Aucubin that was isolated from the plant material was analyzed by Fourier-transform infrared spectroscopy (FTIR) and LC-MS, respectively [70]. Each TLC-isolated compound exhibited a single spot on the HPTLC plate, which confirms an idea about the purity of the isolated compound. Aucubin accompanied by catalpol were determined using LC-MS in different ionization mode. In continuing, many functional groups were recognized in the TLC-isolated aucubin by FTIR [70]. Nevertheless, aucubin and catalpol in Plantago can be quantified by other different methods such as LC-ESI-MS (liquid chromatography-electrospray ionization tandem-mass spectrometry) [62], MPLC [88], LC-TOF-MS (liquid chromatography-time of flight-mass spectrometry) [80], and uHPLC–TOF-MS (ultra-high performance liquid chromatography combined with time-of-flight mass spectrometry) [35]. Various selection programs require rapid and low-cost methods to analyze bioactive components in thousands of samples. FTNIR (Fourier transform near-infrared spectroscopy) supported using chemometric analysis could be a tool to reduce time and costs. A suitable method has been developed to quantify bioactive compounds in plant species [129, 130].
Understanding the mechanism of measuring tools can help us choose the correct and accurate method for measuring compounds (Table 6).

Techniques for determining aucubin and catalpol in pharmacokinetic studies
Although this study has been performed on the methods of determining aucubin and catalpol in Plantago species, mentioning some methods for detecting these compounds in pharmacokinetics can also be useful.
A fast and accurate LC-electro spray ionization (ESI)-MS/MS method has been developed and validated to quantify catalpol in rat plasma [133]. Another group of researchers also validated LC-MS/MS method. However, APCI (atmospheric pressure chemical ionization) was replaced by ESI for the determination of catalpol (m/z of 380/165) in rat plasma and cerebrospinal fluid (CSF) [134]. Therefore, LC-MS/MS was validated as a rapid, sensitive, accurate, and robust method and applied for quantifying aucubin, a main bioactive component of P. asiatica, in rat plasma [135, 136]. On the other hand, Xue et al. introduced the LC-ESI-MS/MS method for simultaneously determining aucubin and catalpol in rat plasma [137]. Zhang et al. created a specific and sensitive high-performance liquid chromatography coupled with a tandem mass spectrometric (HPLC-MS/MS) method. The method was developed to simultaneously determine geniposidic acid and aucubin in rat plasma after oral administration of Du-Zhong tea extract [138]. In this regard, the simultaneous determination of catalpol, morroniside, loganin, and acteoside in the plasma of normal and chronic kidney disease rats by ultra-performance liquid chromatography coupled with mass spectrometry (UPLC-MS) was developed to investigate the combined medicinal extract of R. glutinosa and Cornus officinalis Sieb [139]. Hu et al. presented a selective, sensitive, and efficient ultra-performance liquid chromatography-tandem mass spectrometry (UPLC-MS/MS) method for simultaneously determining 5 active substances, including aucubin in male and female rat plasma after oral administration with Eucommiae cortex extract [140]. Lian et al. developed a novel method for aucubin determination in rat serum with type 1 diabetes using UPLC-MS/MS with supramolecular solvent (SUPRAS)-based on dispersive liquid-liquid microextraction. In general, regarding instrumental analysis, UPLC-MS combines the resolution capability of chromatography with the benefits of speed, specificity, and sensitivity acquired by mass spectrometry (MS). The analytical community has widely admitted this hyphenated technique as a common tool in pharmacokinetic studies [141]. The UHPLC-MS/MS method for aucubin determination exhibited low limits of quantification, good linearity, high extraction recoveries, acceptable accuracy, precision, and stability, in plasma and tissue samples of mice [142].
Biological Activities of Iridoid Glycosides
Iridoids, including secoiridoids, glucosides, esters, and aglycones derivatives, have been reported for medicinal applications [143]. Iridoids are classified as dietary supplements, medicinal foods, and drugs. Iridoids or iridoid-rich plants have established the following biological activities in vitro, in vivo, and clinical research [143]. Iridoids act as a defense for specified plant species and cause various medicinal effects in animals [144]. A large number of iridoids isolated from plants used in traditional medicine have shown various biological activities, thus validating their popular use all over the world. A broad range of biological activities has been reported for iridoids which include anti-diabetic, anti-cancer, anti-inflammatory, anti-microbial, anti-bacterial, anti-oxidant, anti-spasmodic, hepatoprotective, hypolipidemic, hypoglycemic, cardioprotective, choleretic, neuroprotective, purgative, molluscicidal, immunomodulatory, stimulation of bile acid excretion, hepatic dysfunction, anti-tumor, antidotal activities for noxious Amanita mushroom poisoning and anti-viral effects against hepatitis B virus [18, 145-153]. Besides, some iridoids have exhibited anti-protozoal effects against Plasmodium spp. [154, 155], Trypanosoma spp. [156], and Leishmania spp. Indeed, the anti-leishmania activity of iridoids has drawn the scientific community’s attention for decades [157, 158].
Pharmaceutical properties of aucubin and catalpol
Most iridoids, including aucubin and catalpol, have been reported to exhibit significant medicinal properties, such as anti-inflammatory [159], anti-cancer and anti-bacterial activities in vitro and in vivo assay systems. The allure of aucubin and catalpol as cosmetic ingredients in hydrogel formulations was obvious, especially when iridoid glycosides were used as lipid nanoparticles [10]. However, several properties have been reported for the compounds aucubin and catalpol, and understanding the mechanism of action of many of these properties requires more extensive studies.
The study on aucubin confirmed that it possesses extensive pharmacological effects, including anti-aging, anti-bacterial (Table 7), anti-cancer, anti-fibrotic, anti-oxidant, anti-osteoporosis, anti-toxic, neurotrophic, neuroprotective, hepatoprotective, osteoprotective, anti-inflammatory properties as like suppressing the inflammatory act produced by the injection of carrageenan, and healing of skin wounds as like local treatment of oral wounds [152, 160-162].

Aucubin established a significant protective effect against ramanitin intoxication in mice [167]. Aucubin also stimulates the elimination of uric acid from tissues to the blood and the repulsion of uric acid from the kidneys [168]. Aucubin acts as a particular inhibitor of NF-kB (nuclear factor NF-kappa B) in mast cells, which may effectively remedy chronic allergic conditions [169].
Aucubin, and to a greater extent catalpol, are deterrents or toxic to generalists [3, 170]. These compounds apply as oviposition and feeding stimulants for some specialist herbivores [43, 58, 171, 172].
Catalpol has been assessed widely for its biological properties in vitro and in vivo [18]. Although catalpol is more toxic to generalist herbivores than aucubin [3, 43, 170, 173], the research on its properties has shown various pharmacological effects, including sedative, anti-inflammatory, analgesic, anti-tumor, liver protective, anti-microbial (Table 7), purgative, anti-apoptosis actions, and anti-catarrhal for the upper and lower respiratory tract [174-176]. In addition to the listed activities, catalpol has been confirmed as a significant neuroprotective agent against experimental Alzheimer and Parkinson diseases. Catalpol has shown a potential glucose-lowering activity in experimental type 1 and type 2 diabetes mellitus. These activities may be due to improved glucose use in insulin-sensitive tissues and cured mitochondrial biogenesis/function. In addition, catalpol has shown potentially beneficial results in experimental diabetic complications. The significant protective effect of catalpol on cardiovascular was also confirmed. However, in experimental models, catalpol was effective against asthma, hepatotoxicity, and ovarian failure [18].
Anti-cancer properties of aucubin and catalpol
The researchers reported that aucubin inhibited the proliferation of A549 human non-small lung cancer cells by upregulating the expression of p21 and p53 proteins to prevent cell cycle progression in the G0/G1 phase. However, hydrolyzed aucubin showed better anti-leukemia activity than aucubin [177]. Catalpol significantly affected various cancer models, including lung, breast, stomach, and colorectal cancers. One placebo-controlled clinical study confirmed the effect of catalpol on colorectal cancer, which seems to have an anti-cancer impact due to the reduction of inflammation, apoptosis, angiogenesis, and cell cycle arrest [18].
The researchers’ findings show that aucubin and catalpol are known as potential cancer treatments due to their ability to prevent cancer progression and metastasis and induce the death of cancer cells. Therefore, in the present study, some of the properties of these compounds were briefly described. However, anti-cancer properties have been reported in detail (Table 8).

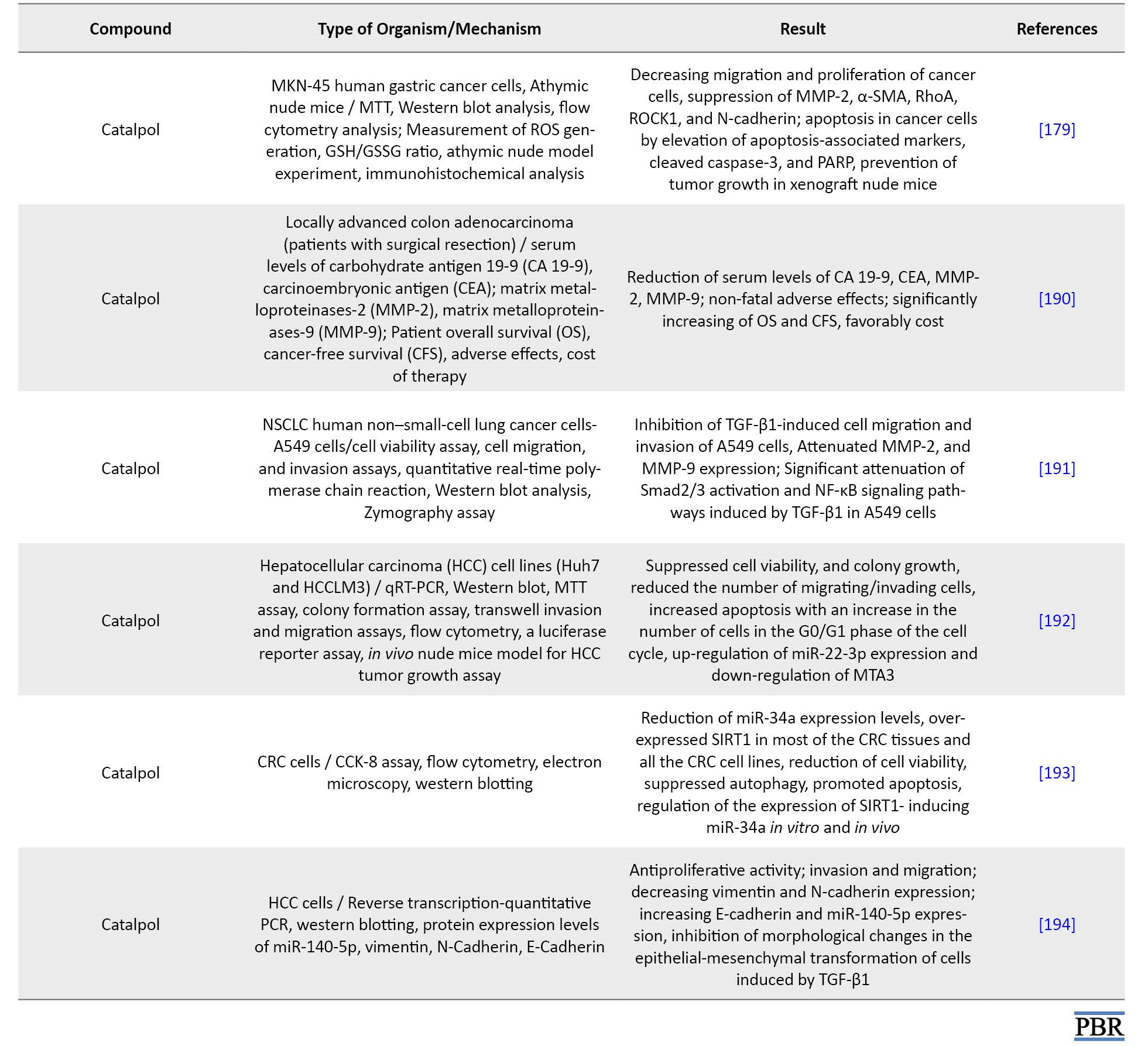
Conclusion
The iridoid patterns exhibited a significant correlation with morphological and other chemical specifications of the representatives of the genus Plantago. Aucubin has extensively been detected in wild plants and especially in P. asiatica. It was reported that aucubin has only been extracted from plants. However, pure products can barely be obtained due to the unstable structure of aucubin. In some studies on Plantago species, aucubin was also found to be more frequently exist compared to catalpol, which could be related to the fact that aucubin is a biosynthetic precursor of catalpol. However, the catalpol value was also observed to be more compared to aucubin content in some Plantago species (P. lanceolata, P. altissima, P. lagopus, and P. argentea) where both iridoids were existence. As a result, leaf age, plant genotype, seasonal changes, and environmental factors affected these variables and influenced the iridoid glycoside concentrations.
The iridoid glycosides, i.e., aucubin and catalpol, are active components with wide pharmacological activities. These compounds have anti-microbial, anti-oxidative, anti-inflammatory, and anti-fungal properties. Therefore, aucubin and catalpol are compounds with abundant sources, good safety, and various biological effects, which demonstrate high value in pharmaceuticals and deserve further research and development.
Aucubin and catalpol have been identified as biologically active in the Plantago species. Furthermore, aucubin and catalpol play many important roles in the medicinal effects of Plantago species, including their hepatoprotective, spasmolytic, collagen synthesis promoting effects, pancreas-protective, neuroprotective, anti-atherogenic, and anti-arthritic. These results suggest Plantago species and their metabolites may apply to human health beyond their traditional uses.
Ethical Considerations
Compliance with ethical guidelines
There were no ethical considerations to be considered in this research.
Funding
This research did not receive any grant from funding agencies in the public, commercial, or non-profit sectors.
Acknowledgments
The author is thankful to the Zanjan University of Medical Sciences and University of Zanjan.
References
- Li YS, Matsunaga K, Ishibashi M, Ohizumi Y. Littoralisone, a novel neuritogenic iridolactone having an unprecedented heptacyclic skeleton including four-and nine-membered rings consisting of glucose from Verbena littoralis.J Org Chem. 2001; 66(6):2165-7. [DOI:10.1021/jo001460d] [PMID]
- Suomi J, Sirén H, Hartonen K, Riekkola ML. Extraction of iridoid glycosides and their determination by micellar electrokinetic capillary chromatography. J Chromatogr A. 2000; 868(1):73-83. [DOI:10.1016/S0021-9673(99)01170-X] [PMID]
- Bowers MD. Iridoid glycosides. In: Rosenthal GA, Berenbaum MR, editors. Herbivores: Their interactions with secondary plant metabolites. New York: Academic Press; 1991. [DOI:10.1016/B978-0-12-597183-6.50013-9]
- Wink M. Evolution of secondary metabolites from an ecological and molecular phylogenetic perspective. Phytochemistry. 2003; 64(1):3-19. [DOI:10.1016/S0031-9422(03)00300-5] [PMID]
- Jensen HF, Jensen SR, Nielsen BJ. Chemotaxonomy of the acanthaceae. Iridoids and quaternary amines. Phytochemistry. 1988; 27(8):2581-9. [DOI:10.1016/0031-9422(88)87029-8]
- Inouye H, Takeda Y, Nishimura H, Kanomi A, Okuda T, Puff C. Chemotaxonomic studies of rubiaceous plants containing iridoid glycosides. Phytochemistry. 1988; 27(8):2591-8. [DOI:10.1016/0031-9422(88)87030-4]
- Junior P. Recent developments in the isolation and structure elucidation of naturally occurring iridoid compounds. Planta Med. 1990; 56(01):1-13. [PMID]
- Andrzejewska-Golec E. The occurrence of iridoids in plants. Acta Soc Bot. Pol. 1995; 64(2):181-6. [DOI:10.5586/asbp.1995.026]
- Drewes SE, Horn MM, Connolly JD, Bredenkamp B. Enolic iridolactone and other iridoids from Alberta magna. Phytochemistry. 1998; 47(6):991-6. [DOI:10.1016/S0031-9422(98)80059-9] [PMID]
- Dąbrowska M, Nowak I. Lipid nanoparticles loaded with selected iridoid glycosides as effective components of hydrogel formulations. Materials. 2021; 14(15):4090. [DOI:10.3390/ma14154090] [PMID] [PMCID]
- Franzyk H. Synthetic aspects of iridoid chemistry. Fortschr Chem Org Naturst. 2000; 79:1-114. [PMID]
- Jensen SR, Schripsema J. Chemotaxonomy and pharmacology of Gentianaceae. In: Struwe L, Albert V, editors. Gentianaceae - systematics and natural history. Cambridge: Cambridge University Press; 2002. [Link]
- Arraché Gonçalves G, Eifler-Lima VL, von Poser GL. Revisiting nature: A review of iridoids as a potential antileishmanial class. Phytochem Rev. 2022; 21(1):101-26. [DOI:10.1007/s11101-021-09750-8] [PMID] [PMCID]
- Jensen SR. Systematic implications of the distribution of iridoids and other chemical compounds in the Loganiaceae and other families of the Asteridae. Ann Mo Bot Gard. 1992; 79(2):284-302. [DOI:10.2307/2399770]
- Rønsted N, Göbel E, Franzyk H, Jensen SR, Olsen CE. Chemotaxonomy of Plantago. Iridoid glucosides and caffeoyl phenylethanoid glycosides. Phytochemistry. 2000; 55(4):337-48. [DOI:10.1016/S0031-9422(00)00306-X] [PMID]
- Trim AR, Hill R. The preparation and properties of aucubin, asperuloside and some related glycosides. Biochem. J. 1952; 50(3):310-9. [PMID] [PMCID]
- Damtoft S. Biosynthesis of catalpol. Phytochemistry. 1994; 35(5):1187-9. [DOI:10.1016/S0031-9422(00)94819-2]
- Bhattamisra SK, Yap KH, Rao V, Choudhury H. Multiple biological effects of an iridoid glucoside, catalpol, and its underlying molecular mechanisms. Biomolecules. 2019; 10(1):32. [PMID] [PMCID]
- Box LA, Judson HG. The concentration of bioactive compounds in Plantago lanceolata is genotype specific. J New Zealand Grasslands. 2018; 80:113-8. [DOI:10.33584/jnzg.2018.80.334]
- Taskova RM, Gotfredsen CH, Jensen SR. Chemotaxonomy of Veroniceae and its allies in the Plantaginaceae. Phytochemistry. 2006; 67(3):286-301. [DOI:10.1016/j.phytochem.2005.11.011] [PMID]
- Gardiner CA, Clough TJ, Cameron KC, Di HJ, Edwards GR, De Klein CA. Potential for forage diet manipulation in New Zealand pasture ecosystems to mitigate ruminant urine derived N2O emissions: A review. New Zealand J Agric Res. 2016; 59(3):301-17. [DOI:10.1080/00288233.2016.1190386]
- Rønsted N, Franzyk H, Mølgaard P, Jaroszewski JW, Jensen SR. Chemotaxonomy and evolution of Plantago L. Plant Syst Evol. 2003; 242:63-82. [DOI:10.1007/s00606-003-0057-3]
- Teramura AH. Experimental ecological genetics in Plantago IX. Differences in growth and vegetative reproduction in Plantago lanceolata L.(Plantaginaceae) from adjacent habitats. Am J Bot. 1983; 70(1):53-8. [DOI:10.1002/j.1537-2197.1983.tb12431.x] [PMID]
- Bos M, Harmens H, Vrieling K. Gene flow in Plantago I. Gene flow and neighbourhood size in P. lanceolata. Heredity. 1986; 56(1):43-54. [DOI:10.1038/hdy.1986.7]
- Bowers MD, Stamp NE. Chemical variation within and between individuals of Plantago lanceolata (Plantaginaceae). J Chem Ecol. 1992; 18(7):985-95. [PMID]
- Bowers MD, Stamp NE. Effects of plant age, genotype and herbivory on Plantago performance and chemistry. Ecology. 1993; 74(6):1778-91. [DOI:10.2307/1939936]
- Deane Bowers M, Collinge SK, Gamble SE, Schmitt J. Effects of genotype, habitat, and seasonal variation on iridoid glycoside content of Plantago lanceolata (Plantaginaceae) and the implications for insect herbivores. Oecologia. 1992; 91(2):201-7. [PMID]
- Fajer ED, Bowers MD, Bazzaz FA. The effect of nutrients and enriched CO2 environments on production of carbon-based allelochemicals in Plantago: A test of the carbon/nutrient balance hypothesis. Am Nat. 1992; 140(4):707-23. [DOI:10.1086/285436] [PMID]
- Van Tienderen PH. Variation in a population of Plantago lanceolata along a topographical gradient. Oikos. 1992; 64(3):560-72. [DOI:10.2307/3545176]
- Stamp NE, Deane Bowers M. Effects of cages, plant age and mechanical clipping on plantain chemistry. Oecologia. 1994; 99(1):66-71. [PMID]
- Gange AC, West HM. Interactions between arbuscular mycorrhizal fungi and foliar-feeding insects in Plantago lanceolata L. New Phytol. 1994; 128(1):79-87. [DOI:10.1111/j.1469-8137.1994.tb03989.x] [PMID]
- Adler LS, Schmitt J, Bowers MD. Genetic variation in defensive chemistry in Plantago lanceolata (Plantaginaceae) and its effect on the specialist herbivore Junonia coenia (Nymphalidae). Oecologia. 1995; 101(1):75-85. [PMID]
- Darrow K, Bowers MD. Phenological and population variation in iridoid glycosides of Plantago lanceolata (Plantaginaceae). Biochem Syst Ecol. 1997; 25(1):1-11. [DOI:10.1016/S0305-1978(96)00090-7]
- Tamura Y, Nishibe S. Changes in the concentrations of bioactive compounds in plantain leaves. J Agric Food Chem. 2002; 50(9):2514-8. [PMID]
- Pankoke H, Buschmann T, Müller C. Role of plant β-glucosidases in the dual defense system of iridoid glycosides and their hydrolyzing enzymes in Plantago lanceolata and Plantago major. Phytochemistry. 2013; 94:99-107. [DOI:10.1016/j.phytochem.2013.04.016] [PMID]
- Tamura Y. [Effects of temperature, light intensity, and nitrogen fertilization on the accumulation of functional components in the herb plantago lanceolata L. (Cultivars and Genetic Resources) (Chinese)]. J Crop Sci Soc Japan. 2001; 70(4):548-53. [DOI:10.1626/jcs.70.548]
- Darrow K, Bowers MD. Effects of herbivore damage and nutrient level on induction of iridoid glycosides in Plantago lanceolata.J Chem Ecol. 1999; 25(6):1427-40. [DOI:10.1023/A:1020991229072]
- Stamp NE, Bowers MD. Do enemies of herbivores influence plant growth and chemistry? Evidence from a seminatural experiment. J Chem Ecol. 2000; 26(10):2367-86. [Link]
- Lampert EC, Bowers MD. Host plant species affects the quality of the generalist Trichoplusia ni as a host for the polyembryonic parasitoid Copidosoma floridanum. Entomol Exp Appl. 2010; 134(3):287-95. [DOI:10.1111/j.1570-7458.2009.00956.x]
- Jarzomski CM, Stamp NE, Bowers MD. Effects of plant phenology, nutrients and herbivory on growth and defensive chemistry of plantain, Plantago lanceolata. Oikos. 2000; 88(2):371-9. [DOI:10.1034/j.1600-0706.2000.880216.x]
- De Deyn GB, Biere A, van der Putten WH, Wagenaar R, Klironomos JN. Chemical defense, mycorrhizal colonization and growth responses in Plantago lanceolata L. Oecologia. 2009; 160(3):433-42. [PMID]
- Nieminen M, Suomi J, Van Nouhuys S, Sauri P, Riekkola ML. Effect of iridoid glycoside content on oviposition host plant choice and parasitism in a specialist herbivore. J Chem Ecol. 2003; 29(4):823-44. [PMID]
- Bowers MD. The evolution of unpalatability and the cost of chemical defense in insects M. Deane Bowers University of Colorado, Boulder. In: Isman MB, Roitberg BD, editors. Insect chemical ecology: An evolutionary approach. New York: Springer US; 1992. [Link]
- Zhu F, Heinen R, van der Sluijs M, Raaijmakers C, Biere A, Bezemer TM. Species-specific plant-soil feedbacks alter herbivore-induced gene expression and defense chemistry in Plantago lanceolata. Oecologia. 2018; 188(3):801-11. [PMID] [PMCID]
- Puffe D, Zerr W. The content of various secondary plant products in widespread weeds in grassland with particular regard to higher lying areas. Eichhof Berichte. 1989; 11:127. [Link]
- Stamp NE, Bowers MD. Effect of temperature and leaf age on growth versus moulting time of a generalist caterpillar fed plantain (Plantago lanceolata). Ecol Entomol. 1994; 19(2):199-206. [DOI:10.1111/j.1365-2311.1994.tb00410.x]
- Jurisić R, Debeljak Z, Vladimir-Knezević S, Vuković J. Determination of aucubin and catalpol in Plantago species by micellar electrokinetic chromatography. Z. Naturforsch. C. 2004; 59(1-2):27-31. [DOI:10.1515/znc-2004-1-206] [PMID]
- Quintero C, Bowers MD. Changes in plant chemical defenses and nutritional quality as a function of ontogeny in Plantago lanceolata (Plantaginaceae). Oecologia. 2012; 168(2):471-81. [PMID]
- Pellissier L, Roger A, Bilat J, Rasmann S. High elevation Plantago lanceolata plants are less resistant to herbivory than their low elevation conspecifics: Is it just temperature?. Ecography. 2014; 37(10):950-9. [DOI:10.1111/ecog.00833]
- Marak HB, Biere A, Damme JM. Fitness costs of chemical defense in Plantago lanceolata L.: Effects of nutrient and competition stress. Evolution. 2003; 57(11):2519-30. [DOI:10.1111/j.0014-3820.2003.tb01496.x]
- Reudler JH, Biere A, Harvey JA, Van Nouhuys S. Differential performance of a specialist and two generalist herbivores and their parasitoids on Plantago lanceolata. J Chem Ecol. 2011; 37(7):765-78. [PMID] [PMCID]
- Navarrete S, Kemp PD, Pain SJ, Back PJ. Bioactive compounds, aucubin and acteoside, in plantain (Plantago lanceolata L.) and their effect on in vitro rumen fermentation. Anim Feed Sci Technol. 2016; 222:158-67. [DOI:10.1016/j.anifeedsci.2016.10.008]
- Bowers MD. Variation in iridoid glycosides in a population of Plantago patagonica Jacq.(Plantaginaceae) in Colorado. Biochem Syst Ecol. 1996; 24(3):207-10. [DOI:10.1016/0305-1978(96)00022-1]
- Willinger G, Dobler S. Selective sequestration of iridoid glycosides from their host plants in Longitarsus flea beetles. Biochem Syst Ecol. 2001; 29(4):335-46. [DOI:10.1016/S0305-1978(00)00082-X] [PMID]
- Marak HB, Biere A, Van Damme JM. Systemic, genotype-specific induction of two herbivore-deterrent iridoid glycosides in Plantago lanceolata L. in response to fungal infection by Diaporthe adunca (Rob.) Niessel. J Chem Ecol. 2002; 28(12):2429-48. [Link]
- Biere A, Marak HB, van Damme JM. Plant chemical defense against herbivores and pathogens: Generalized defense or trade-offs? Oecologia. 2004; 140(3):430-41. [PMID]
- Harvey JA, Van Nouhuys S, Biere A. Effects of quantitative variation in allelochemicals in Plantago lanceolata on development of a generalist and a specialist herbivore and their endoparasitoids. J Chem Ecol. 2005; 31(2):287-302. [PMID]
- Reudler Talsma JH, Biere A, Harvey JA, van Nouhuys S. Oviposition cues for a specialist butterfly-plant chemistry and size. J Chem Ecol. 2008; 34(9):1202-12. [PMID] [PMCID]
- AL-Mamunm M, Abe D, Kofujita H, Tamura Y, Sano H. Comparison of the bioactive components of the ecotypes and cultivars of plantain (Plantago lanceolata L.) herbs. Anim Sci J. 2008; 79(1):83-8. [DOI:10.1111/j.1740-0929.2007.00501.x]
- Kim BH, Lee NK, Chang IM. Simultaneous analysis of a mixture of iridoids in water extracts of plantago asiatica L. by LC. Chromatographia. 2009; 69(11):1397-400. [Link]
- Gonda S, Tóth L, Parizsa P, Nyitrai M, Vasas G. Screening of common Plantago species in Hungary for bioactive molecules and antioxidant activity. Acta Biol Hung. 2010; 61 Suppl:25-34. [PMID]
- Janković T, Menković N, Zdunić G, Beara I, Balog K, Šavikin K, et al. Quantitative determination of aucubin in seven plantago species using hplc, hptlc, and lc-esi-ms methods. Anal Lett. 2010; 43(16):2487-95. [DOI:10.1080/00032711003725581]
- Kartini K, Azminah A. Chromatographic fingerprinting and clustering of Plantago major L. from different areas in Indonesia. Asian J Pharm Clin Res. 2012; 5(4):191-5. [Link]
- Dietz M, Machill S, Hoffmann HC, Schmidtke K. Inhibitory effects of Plantago lanceolata L. on soil N mineralization. Plant Soil. 2013; 368(1):445-58. [Link]
- Gonda S, Nguyen NM, Batta G, Gyémánt G, Máthé C, Vasas G. Determination of phenylethanoid glycosides and iridoid glycosides from therapeutically used Plantago species by CE-MEKC. Electrophoresis. 2013; 34(17):2577-84. [PMID]
- Miehe-Steier A, Roscher C, Reichelt M, Gershenzon J, Unsicker SB. Light and nutrient dependent responses in secondary metabolites of Plantago lanceolata offspring are due to phenotypic plasticity in experimental grasslands. PLoS One. 2015; 10(9):e0136073. [DOI:10.1371/journal.pone.0136073] [PMID] [PMCID]
- Sarkar S, Lal RK, Verma RK. Quantification of β-carotene, aucubin content, their associations and contribution to other economic traits in Plantago germplasm. Trends Phytochem. Res. 2017; 1(4):215-26. [Link]
- Kalantari A, Kósa D, Nemes D, Ujhelyi Z, Fehér P, Vecsernyés M, et al. Self-nanoemulsifying drug delivery systems containing Plantago lanceolata-an assessment of their antioxidant and antiinflammatory effects. Molecules. 2017; 22(10):1773. [PMID] [PMCID]
- Box LA, Edwards GR, Bryant RH. Seasonal and diurnal changes in aucubin, catalpol and acteoside concentration of plantain herbage grown at high and low N fertiliser inputs. New Zealand J Agric Res. 2019; 62(3):343-53. [DOI:10.1080/00288233.2018.1505641]
- Hasan AK, Ibrahim SA, Amani AT, Monther FM. Determination, isolation, and identification of aucubin and verbascoside in the leaves of Iraqi Plantago lancoleta L. using different detecting methods. Int J Pharm Phamaceut Sci. 2018; 11(2):74-80. [DOI:10.22159/ijpps.2019v11i2.30566]
- Majkić T, Bekvalac K, Beara I. Plantain (Plantago L.) species as modulators of prostaglandin E2 and thromboxane A2 production in inflammation. J Ethnopharmacol. 2020; 262:113140. [PMID]
- Suh NJ, Shim CK, Lee MH, Kim SK, Chang IM. Pharmacokinetic study of an iridoid glucoside: Aucubin. Pharm Res. 1991; 8(8):1059-63. [PMID]
- Bianco A. The chemistry of iridoids. ChemInform. 1991; 22(19). [Link]
- Inouye HI. Iridoids. Methods in Plant Biochemistry. 1991; 7:99-143. [Link]
- Li H, Hu J, Ouyang H, Li Y, Shi H, Ma C, et al. Extraction of aucubin from seeds of Eucommia ulmoides Oliv. Using supercritical carbon dioxide. J AOAC Int. 2009; 92(1):103-10. [DOI:10.1093/jaoac/92.1.103] [PMID]
- Camps C, Simonnet X, Dubourdeaux M. FT NIR assisted Machine and Deep learning for determination of Acteoside, Aucubin and Catalpol contents of Plantago lanceolata. Agric Res Technol. 2020; 25(2):64-8. [Link]
- Gardner DR, Stermitz FR Hostplant utilization and iridoid glycoside sequestration by Euphydryas anicia (Lepidoptera: Nymphalidae). J Chem Ecol. 1988; 14(12):2147-68. [PMID]
- Fajer ED, Bowers MD, Bazzaz FA. The effects of enriched carbon dioxide atmospheres on plant-insect herbivore interactions. Science. 1989; 243(4895):1198-200. [DOI:10.1126/science.243.4895.1198] [PMID]
- Klockars GK, Bowers MD, Cooney B. Leaf variation in iridoid glycoside content of Plantago lanceolata (Plantaginaceae) and oviposition of the buckeye, Junonia coenia (Nymphalidae). Chemoecology. 1993; 4:72-8. [Link]
- Sutter R, Müller C. Mining for treatment-specific and general changes in target compounds and metabolic fingerprints in response to herbivory and phytohormones in Plantago lanceolata. New Phytol. 2011; 191(4):1069-82. [DOI:10.1111/j.1469-8137.2011.03768.x] [PMID]
- Bennett AE, Macrae AM, Moore BD, Caul S, Johnson SN. Early root herbivory impairs arbuscular mycorrhizal fungal colonization and shifts defence allocation in establishing Plantago lanceolata. PLoS One. 2013; 8(6):e66053. [PMID] [PMCID]
- Pankoke H, Gehring R, Müller C. Impact of the dual defence system of Plantago lanceolata (Plantaginaceae) on performance, nutrient utilisation and feeding choice behaviour of Amata mogadorensis larvae (Lepidoptera, Erebidae). J Insect Physiol. 2015; 82:99-108. [DOI:10.1016/j.jinsphys.2015.08.006] [PMID]
- Richards LA, Lampert EC, Bowers MD, Dodson CD, Smilanich AM, Dyer LA. Synergistic effects of iridoid glycosides on the survival, development and immune response of a specialist caterpillar, Junonia coenia (Nymphalidae). J Chem Ecol. 2012; 38(10):1276-84. [PMID]
- Wagner H, Bladt S, Zgainski EM. Bitterstoff-drogen. In: Drogenanalyse. Berlin: Springer; 1983. [Link]
- Andrzejewska Golec E, Swiatek L. Chemotaxonomic studies on the genus Plantago. I. Analysis of the iridoid fraction. Herba Pol. 1984; 30: 9-16. [Link]
- Kuzmanov BA, Evstatieva LN, Marekov NL, Popov SS. Chemosystematic study on genus Plantago L. Fitologiia. 1984; 24:29-34. [Link]
- Taskova R, Evstatieva L, Handjieva N, Popov S. Iridoid patterns of genus Plantago L. and their systematic significance. Z Naturforsch C J Biosci. 2002; 57(1-2):42-50. [DOI:10.1515/znc-2002-1-208] [PMID]
- Genç Y, Saraçoğlu İ, Nagatsu A, Harput ÜŞ. Iridoid and megastigman glucosides from Plantago lagopus L. Fabad J Pharm Sci. 2010; 35:29-34. [Link]
- Rahn K. A phylogenetic study of the Plantaginaceae. Bot J Linn Soc. 1996; 120(2):145-98. [DOI:10.1111/j.1095-8339.1996.tb00484.x]
- Handjieva N, Saadi H, Evstatieva L. Iridoid glueosides from Plantago altissima L., Plantago lanceolata L., Plantago atrata hoppe and Plantago argentea Chaix. Z. Naturforsch C. 2014; 46(11-12):963-5. [DOI:10.1515/znc-1991-11-1206]
- Afifi MS, Zaghloul MG, Hassan MA. Phytochemical investigation of the aerial parts of Plantago ovata. Mansouri J Pharm Sci. 2000; 16(2):178-90. [Link]
- Saadi H, Handjieva N, Ivanova A, Popov S. Iridoids from Plantago carinata Schrad. Z Naturforsch C. 1991; 46(11-12):1001-2. [DOI:10.1515/znc-1991-11-1212]
- Andrzejewska-Golec E. Iridoid glucosides in Littorella uniflora (L.) Ascherson. Acta Soc. Bot Pol. 1999; 68(4):267-8. [DOI:10.5586/asbp.1999.036]
- Jensen SR, Olsen CE, Rahn K, Rasmussen JH. Iridoid glucosides in Plantago alpina and P. Altissima. Phytochemistry. 1996; 42(6):1633-6. [DOI:10.1016/0031-9422(96)00158-6]
- Oshio H, Inouye H. Two new iridoid glucosides of Plantago asiatica. Planta Med. 1982; 44(4):204-6. [PMID]
- Endo T, Taguchi H, Yosioka I. The glycosides of plantago major var. Japonica Nakai. A new flavanone glycoside, plantagoside. Chem Pharm Bull. 1981; 29(4):1000-4. [DOI:10.1248/cpb.29.1000]
- Handjieva N, Taskova R, Popov S. 10-Hydroxymajoroside, an iridoid glucoside from Plantago cornuti Gouan L. Z Naturforsch C. 1993; 48(11-12):827-8. [DOI:10.1515/znc-1993-11-1202]
- Damtoft S, Falkesgaard E, Jensen SR. Iridoid glucosides from Plantago hookeriana. Phytochemistry. 1994; 35(5):1367-8. [DOI:10.1016/S0031-9422(06)80127-5]
- Afifi MS, Salema OM, Maatooq GT. Phytochemical study of two plantago species: Part II: Iridoid glucosides. Mansoura J Pharm Sci. 1990; 6(4):16-25. [Link]
- Long C, Moulis C, Stanislas E, Fouraste I. [L’aucuboside et le catalpol dans les feuilles de Plantago lanceolata L., Plantago major L. et Plantago media L (French)]. J Pharm Belg. 1995; 50(6):484-8. [Link]
- Marak HB, Biere A, Van Damme JM. Direct and correlated responses to selection on iridoid glycosides in Plantago lanceolata L. J Evol Biol. 2000; 13(6):985-96. [DOI:10.1046/j.1420-9101.2000.00233.x]
- Barton KE. Early ontogenetic patterns in chemical defense in Plantago (Plantaginaceae): Genetic variation and trade-offs. Am J Bot. 2007; 94(1):56-66. [DOI:10.3732/ajb.94.1.56] [PMID]
- Wurst S, Wagenaar R, Biere A, Van der Putten WH. Microorganisms and nematodes increase levels of secondary metabolites in roots and root exudates of Plantago lanceolata. Plant Soil. 2010; 329:117-26. [Link]
- Mraja A, Unsicker SB, Reichelt M, Gershenzon J, Roscher C. Plant community diversity influences allocation to direct chemical defence in Plantago lanceolata. PLoS One. 2011; 6(12):e28055. [DOI:10.1371/journal.pone.0028055] [PMID] [PMCID]
- Navrátilová M, Raisová Stuchlíková L, Skálová L, Szotáková B, Langhansová L, Podlipná R. Pharmaceuticals in environment: The effect of ivermectin on ribwort plantain (Plantago lanceolata L.). Environ Sci Pollut Res. 2020; 27(25):31202-10. [DOI:10.1007/s11356-020-09442-4] [PMID]
- Rahamooz-Haghighi S, Bagheri K, Sharafi A, Danafar H. Establishment and elicitation of transgenic root culture of Plantago lanceolata and evaluation of its anti-bacterial and cytotoxicity activity. Prep Biochem Biotechnol. 2021; 51(3):207-24. [PMID]
- Rahamouz-Haghighi S, Sharafi A, Bagheri K. In vitro organogenesis from transformed root cultures of Plantago lanceolata and phytochemical analysis by HPLC and GC-MS. J BioSci Biotechnol. 2021; 10(2):87-97. [Link]
- Murai M, Takenaka T, Nishibe S. Iridoids from Plantago major. J Nat Med. 1996; 50(4):306. [Link]
- Rahamouz-Haghighi S, Bagheri K, Sharafi A. In vitro elicitation and detection of apigenin, catalpol and gallic acid in hairy root culture of Plantago major L. and assessment of cytotoxicity and anti-bacterial activity of its methanolic extract. Nat Prod Res. 2023; 37(4):633-637. [PMID]
- Świątek L. The iridoid phenolic acids and glycosides in certain Polish medicinal herbs from the Plantago genus. Herba Polonica. 1977; 23:201-10. [Link]
- Saadi H, Handjieva N, Popov S, Evstatievat L. Iridoids from Plantago media. Phytochemistry. 1990; 29(12):3938-9. [DOI:10.1016/0031-9422(90)85366-N]
- Franzyk H, Husum TL, Jensen SR. A caffeoyl phenylethanoid glycoside from Plantago myosuros. Phytochemistry. 1998; 47(6):1161-2. [DOI:10.1016/S0031-9422(98)80092-7]
- Johnston J, Bowers MD, Ranker TA. Iridoid glycoside and allozyme variation within and among populations of Plantago rhodosperma Decne.(Plantaginaceae). Biochem Syst Ecol. 1997; 25(7):581-90. [DOI:10.1016/S0305-1978(97)00040-9]
- Li H, Chen B, Zhang Z, Yao S. Focused microwave-assisted solvent extraction and HPLC determination of effective constituents in Eucommia ulmodies Oliv.(E. ulmodies). Talanta. 2004; 63(3):659-65. [DOI:10.1016/j.talanta.2003.12.028] [PMID]
- Gonda S, Tóth L, Gyémánt G, Braun M, Emri T, Vasas G. Effect of high relative humidity on dried Plantago lanceolata L. leaves during long-term storage: Effects on chemical composition, colour and microbiological quality. Phytochem Anal. 2012; 23(1):88-93. [DOI:10.1002/pca.1329] [PMID]
- Miething H, Holz W, Hänsel R. HPLC-Gehaltsbestimmung von aucubin in drogen und zubereitungen. Pharm Ztg. 1986; 131:746-7. [Link]
- Rischer M, Adamczyk M, Ratz H, Hose S, Marchesan M, Franz G, et al. Quantitative determination of the iridoid glycosides aucubin and catalpol in Plantago lanceolata L. extracts by HPTLC and HPLC. J Plan Chrom. 1998; 11:374-8. [Link]
- Biringanine G, Chiarelli MT, Faes M, Duez P. A validation protocol for the HPTLC standardization of herbal products: Application to the determination of acteoside in leaves of Plantago palmata Hook. fs. Talanta. 2006; 69(2):418-24. [DOI:10.1016/j.talanta.2005.10.007] [PMID]
- Marak HB, Biere A, Van Damme JM. Two herbivore-deterrent iridoid glycosides reduce the in-vitro growth of a specialist but not of a generalist pathogenic fungus of Plantago lanceolata L. Chemoecology. 2002; 12(4):185-92. [Link]
- Qu L, Wang M, Biere A. Interactive effects of mycorrhizae, soil phosphorus, and light on growth and induction and priming of defense in Plantago lanceolata. Front Plant Sci. 2021; 12:647372. [DOI:10.3389/fpls.2021.647372] [PMID] [PMCID]
- Kim BH, Park KS, Chang IM. Elucidation of anti-inflammatory potencies of Eucommia ulmoides bark and Plantago asiatica seeds. J Med Food. 2009; 12(4):764-9. [DOI:10.1089/jmf.2008.1239] [PMID]
- Gardiner CA, Clough TJ, Cameron KC, Di HJ, Edwards GR, de Klein CA. Potential inhibition of urine patch nitrous oxide emissions by Plantago lanceolata and its metabolite aucubin. New Zealand J Agric Res. 2018; 61(4):495-503. [DOI:10.1080/00288233.2017.1411953]
- Li Y, Wang MJ, Zhao Y, Sun WJ. Analysis of conversion of aucubin into aucubigenin by emulsin. Chin J Pharm Anal. 2008; 28(12):2001-4. [Link]
- Hong JL, Qin XY, Shu P, Wu G, Wang Q, Qin MJ. Analysis of catalpol derivatives by characteristic neutral losses using liquid chromatography combined with electrospray ionization multistage and time-of-flight mass spectrometry. Rapid Commun Mass Spectrom. 2010; 24(17):2680-6. [PMID]
- Chankvetadze B. Enantioseparations by using capillary electrophoretic techniques. The story of 20 and a few more years. J Chromatogr A. 2007; 1168(1-2):45-70. [DOI:10.1016/j.chroma.2007.08.008] [PMID]
- Wu HK, Chuang WC, Sheu SJ. Separation of nine iridoids by capillary electrophoresis and high-performance liquid chromatography. J Chromatogr A. 1998; 803(1-2):179-87. [DOI:10.1016/S0021-9673(97)01227-2]
- Suomi J, Sirén H, Wiedmer SK, Riekkola ML. Isolation of aucubin and catalpol from Melitaea cinxia larvae and quantification by micellar electrokinetic capillary chromatography. Anal Chim Acta. 2001; 429(1):91-9. [DOI:10.1016/S0003-2670(00)01266-6]
- Suomi J, Wiedmer SK, Jussila M, Riekkola ML. Determination of iridoid glycosides by micellar electrokinetic capillary chromatography-mass spectrometry with use of the partial filling technique. Electrophoresis. 2001; 22(12):2580-7. [PMID]
- Camps C, Gérard M, Quennoz M, Brabant C, Oberson C, Simonnet X. Prediction of essential oil content of oregano by hand-held and Fourier transform NIR spectroscopy. J Sci Food Agric. 2014; 94(7):1397-402. [DOI:10.1002/jsfa.6427] [PMID]
- Camps C, Toussirot M, Quennoz M, Simonnet X. Determination of artemisinin and moisture content of Artemisia annua L. dry powder using a hand-held near infrared spectroscopy device. J Near Infrared Spectrosc. 2011; 19(3):191-8. [DOI:10.1255/jnirs.927]
- De Schutter JA, Van der Weken G, Van den Bossche W, De Moerloose P. On the problem of the purity of solvents in HPTLC. Chromatographia. 1985; 20:739-42. [Link]
- Silva M. Micellar electrokinetic chromatography: A review of methodological and instrumental innovations focusing on practical aspects. Electrophoresis. 2013; 34(1):141-58. [DOI:10.1002/elps.201200349] [PMID]
- Lu R, Gu Y, Si D, Liu C. Quantitation of catalpol in rat plasma by liquid chromatography/electrospray ionization tandem mass spectrometry and its pharmacokinetic study. J Chromatogr B Analyt Technol Biomed Life Sci. 2009; 877(29):3589-94. [DOI:10.1016/j.jchromb.2009.08.047] [PMID]
- Wang HQ, Xu YX, Zhu CQ. Upregulation of heme oxygenase-1 by acteoside through ERK and PI3 K/Akt pathway confer neuroprotection against beta-amyloid-induced neurotoxicity. Neurotoxic Res. 2012; 21(4):368-78. [PMID]
- Xu W, Deng Z, Guo H, Ling P. A rapid and sensitive determination of aucubin in rat plasma by liquid chromatography-tandem mass spectrometry and its pharmacokinetic application. Biomed Chromatogr. 2012; 26(9):1066-70. [DOI:10.1002/bmc.1748] [PMID]
- Huang YX, Er-Wei LI, Lei WA, Yan HU, Qiang WA, Olaleye O, et al. LC/MS/MS determination and pharmacokinetic studies of six compounds in rat plasma following oral administration of the single and combined extracts of Eucommia ulmoides and Dipsacus asperoides. Chin J Nat Med. 2014; 12(6):469-76. [DOI:10.1016/S1875-5364(14)60073-X] [PMID]
- Xue B, Ma B, Zhang Q, Li X, Zhu J, Liu M, et al. Pharmacokinetics and tissue distribution of Aucubin, Ajugol and Catalpol in rats using a validated simultaneous LC-ESI-MS/MS assay. J Chromatogr B Analyt Technol Biomed Life Sci. 2015; 1002:245-53. [DOI:10.1016/j.jchromb.2015.08.026] [PMID]
- Zhang L, Ma YL, Liu Y, Zu YG. Development and validation of high liquid performance chromatography-tandem mass spectrometry method for simultaneous determination of geniposidic acid and aucubin in rat plasma for pharmacokinetic study after oral administration of Du-zhong tea extract. J Chromatogr B Analyt Technol Biomed Life Sci. 2014; 963:62-9. [DOI:10.1016/j.jchromb.2014.04.033] [PMID]
- Zhao M, Tao J, Qian D, Liu P, Shang EX, Jiang S, et al. Simultaneous determination of loganin, morroniside, catalpol and acteoside in normal and chronic kidney disease rat plasma by UPLC-MS for investigating the pharmacokinetics of Rehmannia glutinosa and Cornus officinalis Sieb drug pair extract. J Chromatogr B Analyt Technol Biomed Life Sci. 2016; 1009-1010:122-9. [PMID]
- Hu F, An J, Li W, Zhang Z, Chen W, Wang C, et al. UPLC-MS/MS determination and gender-related pharmacokinetic study of five active ingredients in rat plasma after oral administration of Eucommia cortex extract. J Ethnopharmacol. 2015; 169:145-55. [DOI:10.1016/j.jep.2015.04.007] [PMID]
- Lian X, Wang N, Ma L, Jiang H, Bai D, Xue H, et al. Determination of aucubin by supramolecular solvent-based dispersive liquid-liquid microextraction and UPLC-MS/MS: Application to a pharmacokinetic study in rats with type 1 diabetes. J Pharm Biomed Anal. 2020; 186:113301. [DOI:10.1016/j.jpba.2020.113301] [PMID]
- An J, Hu F, Wang C, Zhang Z, Yang L, Wang Z. Pharmacokinetics and tissue distribution of five active ingredients of Eucommiae cortex in normal and ovariectomized mice by UHPLC-MS/MS. Xenobiotica. 2016; 46(9):793-804. [DOI:10.3109/00498254.2015.1129470] [PMID]
- Preeti A, Dutta D, Yadav R, Rana M, Moudgil S. Systematic studies on iridoids. Int J Pharm Sci Res. 2021; 13(3). [DOI:10.31838/ijpr/2021.13.03.126]
- Li C, Lan M, Lv J, Zhang Y, Gao X, Gao X, et al. Screening of the hepatotoxic components in Fructus Gardeniae and their effects on rat liver BRL-3A cells. Molecules. 2019; 24(21):3920. [DOI:10.3390/molecules24213920] [PMID] [PMCID]
- Kubo I, Matsumoto A. Molluscicides from olive Olea europaea and their efficient isolation by counter-current chromatographies. J Agric Food Chem. 1984; 32(3):687-8. [DOI:10.1021/jf00123a067]
- Drewes SE, Kayonga L, Clark TE, Brackenbury TD, Appleton CC. Iridoid molluscicidal compounds from Apodytes dimidiata. J Nat Prod. 1996; 59(12):1169-70. [DOI:10.1021/np960404y]
- Hamadi HK, Castellon R. Oleuropein, a non-toxic olive iridoid, is an anti-tumor agent and cytoskeleton disrupter. Biochem Biophys Res Commun. 2005; 334(3):769-8. [PMID]
- de Sa Barreto LC, de BCarvalho EF, da Cunha-Filho MS, Ferreira CP, Xavier HS. [Atividade moluscicida de extratos e de aucubina de Vitex gardneriana schauer (Verbenaceae) em embriões da Biomphalaria glabrata (Portuguese)]. Latin Am J Pharm. 2007; 26(3):339-43. [Link]
- Tundis R, Loizzo MR, Menichini F, Statti GA, Menichini F. Biological and pharmacological activities of iridoids: Recent developments. Mini Rev Med Chem. 2008; 8(4):399-420. [DOI:10.2174/138955708783955926] [PMID]
- Awang DV. Tyler’s herbs of choice: The therapeutic use of phytomedicinals. CRC Press: Boca Raton; 2009. [DOI:10.1201/9780789038739]
- Pan Y, Zhao YL, Zhang J, Li WY, Wang YZ. Phytochemistry and pharmacological activities of the genus Gentiana (Gentianaceae). Chem Biodivers. 2016; 13(2):107-50. [DOI:10.1002/cbdv.201500333] [PMID]
- Zeng X, Guo F, Ouyang D. A review of the pharmacology and toxicology of aucubin. Fitoterapia. 2020; 140:104443. [DOI:10.1016/j.fitote.2019.104443] [PMID]
- Wang C, Gong X, Bo A, Zhang L, Zhang M, Zang E, Zhang C, Li M. Iridoids: Research advances in their phytochemistry, biological activities, and pharmacokinetics. Molecules. 2020; 25(2):287. [DOI:10.3390/molecules25020287] [PMID] [PMCID]
- Silva LF, Lima ES, Vasconcellos MC, Aranha ES, Costa DS, Mustafa EV, et al. In vitro and in vivo antimalarial activity and cytotoxicity of extracts, fractions and a substance isolated from the Amazonian plant Tachia grandiflora (Gentianaceae). Mem Inst Oswaldo Cruz. 2013; 108(4):501-7. [PMID] [PMCID]
- Tamura S, Kubata BK, Syamsurizal, Itagaki S, Horii T, Taba MK, et al. New anti-malarial phenylpropanoid conjugated iridoids from Morinda morindoides. Bioorg Med Chem Lett. 2010; 20(5):1520-3. [DOI:10.1016/j.bmcl.2010.01.095] [PMID]
- Kwofie KD, Tung NH, Suzuki-Ohashi M, Amoa-Bosompem M, Adegle R, Sakyiamah MM, et al. Antitrypanosomal activities and mechanisms of action of novel tetracyclic iridoids from Morinda lucida benth. Antimicrob Agents Chemother. 2016; 60(6):3283-90. [DOI:10.1128/AAC.01916-15] [PMID] [PMCID]
- Hussain H, Al-Harrasi A, Al-Rawahi A, Green IR, Gibbons S. Fruitful decade for antileishmanial compounds from 2002 to late 2011. Chem Rev. 2014; 114(20):10369-428. [DOI:10.1021/cr400552x] [PMID]
- Hussain H, Green IR, Saleem M, Raza ML, Nazir M. Therapeutic potential of iridoid derivatives: Patent review. Inventions. 2019; 4(2):29. [DOI:10.3390/inventions4020029]
- Shan M, Yu S, Yan H, Guo S, Xiao W, Wang Z, et al. A review on the phytochemistry, pharmacology, pharmacokinetics and toxicology of geniposide, a natural product. Molecules. 2017; 22(10):1689. [DOI:10.3390/molecules22101689] [PMID] [PMCID]
- Recio MC, Giner RM, Máñez S, Ríos JL. Structural considerations on the iridoids as anti-inflammatory agents. Planta Med. 1994; 60(3):232-4. [PMID]
- Kang Z, Wu WH, Wang JJ, Ouyang DS. [Research advances in pharmacology of aucubin and aucubigenin (Chinese)].Zhonggon Zhong Yao Za Zhi,. 2007; 32(24):2585-7. [PMID]
- Shim KM, Choi SH, Jeong MJ, Kang SS. Effects of aucubin on the healing of oral wounds. In Vivo. 2007; 21(6):1037-41. [PMID]
- Ishiguro K, Yamaki M, Takagi S. [Studies on the iridoid related compounds. I. On the antimicrobial activity of aucubigenin and certain iridoid aglycones (Japanese)]. Yakugaku Zasshi. 1982; 102(8):755-9. [DOI:10.1248/yakushi1947.102.8_755] [PMID]
- Li Y, Chen L, Han G, Zhou J, Zhao Y. Determination of antibacterial activity of aucubigenin and aucubin. Asian J Chem. 2014; 26(2):559-61. [DOI:10.14233/ajchem.2014.15833]
- Shirley KP, Windsor LJ, Eckert GJ, Gregory RL. In vitro effects of Plantago major extract, aucubin, and baicalein on Candida albicans biofilm formation, metabolic activity, and cell surface hydrophobicity. J Prosthodont. 2017; 26(6):508-15. [DOI:10.1111/jopr.12411] [PMID]
- Kahraman Ç, Tatli İİ, Kart D, Ekizoğlu M, Akdemir ZŞ. Structure elucidation and antimicrobial activities of secondary metabolites from the flowery parts of verbascum mucronatum lam. Turkish J Pharm Sci. 2018; 15(3):231-7. [DOI:10.4274/tjps.50479] [PMID] [PMCID]
- Chang LM, Yun HS, Kim YS, Ahn JW. Aucubin: Potential antidote for alpha-amanitin poisoning. J Toxicol Clin Toxicol. 1984; 22(1):77-85. [PMID]
- Kato Y. Mechanism of uric acid excretion stimulation by aucubin. Folia Phamacol. Japon. 1946; 42:27-40. [Link]
- Jeong HJ, Koo HN, Na HJ, Kim MS, Hong SH, Eom JW, et al. Inhibition of TNF-α and IL-6 production by aucubin through blockade of NF-κB activation in RBL-2H3 mast cells. Cytokine. 2002; 18(5):252-9. [DOI:10.1006/cyto.2002.0894] [PMID]
- Puttick GM, Bowers MD. Effect of qualitative and quantitative variation in allelochemicals on a generalist insect: Iridoid glycosides and the southern armyworm. J Chem Ecol. 1988; 14(1):335-51. [PMID]
- Bowers MD. Iridoid glycosides and host-plant specificity in larvae of the buckeye butterfly, Junonia coenia (Nymphalidae). J Chem Ecol. 1984; 10(11):1567-77. [PMID]
- Pereyra PC, Bowers MD. Iridoid glycosides as oviposition stimulants for the buckeye butterfly, Junonia coenia (Nymphalidae). J Chem Ecol. 1988; 14(3):917-28. [PMID]
- Bowers MD, Puttick GM. Fate of ingested iridoid glycosides in lepidopteran herbivores. J Chem Ecol. 1986; 12(1):169-78. [PMID]
- Zhu HF, Wan D, Luo Y, Zhou JL, Chen L, Xu XY. Catalpol increases brain angiogenesis and up-regulates VEGF and EPO in the rat after permanent middle cerebral artery occlusion. Int J Biol Sci. 2010; 6(5):443-53. [DOI:10.7150/ijbs.6.443] [PMID] [PMCID]
- Bone K, Mills S, Mills SY. Principles and practice of phytotherapy: Modern herbal medicine. London: Churchill Livingstone; 2013. [Link]
- Jiang B, Shen RF, Bi J, Tian XS, Hinchliffe T, Xia Y. Catalpol: A potential therapeutic for neurodegenerative diseases. Curr Med Chem. 2015; 22(10):1278-91. [DOI:10.2174/0929867322666150114151720] [PMID]
- Hung JY, Yang CJ, Tsai YM, Huang HW, Huang MS. Antiproliferative activity of aucubin is through cell cycle arrest and apoptosis in human non-small cell lung cancer A549 cells. Clin Exp Pharmacol Physiol. 2008; 35(9):995-1001. [DOI:10.1111/j.1440-1681.2008.04935.x] [PMID]
- Chiang LC, Chiang W, Chang MY, Ng LT, Lin CC. Antileukemic activity of selected natural products in Taiwan. Am J Chin Med. 2003; 31(1):37-46. [DOI:10.1142/S0192415X03000825] [PMID]
- Pungitore CR, León LG, García C, Martín VS, Tonn CE, Padrón JM. Novel antiproliferative analogs of the Taq DNA polymerase inhibitor catalpol. Bioorg Med Chem Lett. 2007; 17(5):1332-5. [DOI:10.1016/j.bmcl.2006.11.086] [PMID]
- García C, León LG, Pungitore CR, Ríos-Luci C, Daranas AH, Montero JC, et al. Enhancement of antiproliferative activity by molecular simplification of catalpol. Bioorg Med Chem. 2010; 18(7):2515-23. [DOI:10.1016/j.bmc.2010.02.044] [PMID]
- Hao Q, Niu J, Wang J, Zhao P. Phytoestrogenic effects of catalpol in T47D and MDA-MB231 cells in culture. FASEB J. 2010; 24(S1):821-9. [DOI:10.1096/fasebj.24.1_supplement.821.9]
- Gao N, Tian JX, Shang YH, Zhao DY, Wu T. Catalpol suppresses proliferation and facilitates apoptosis of OVCAR-3 ovarian cancer cells through upregulating microRNA-200 and downregulating MMP-2 expression. Int J Mol Sci. 2014; 15(11):19394-405. [DOI:10.3390/ijms151119394] [PMID] [PMCID]
- Kim MB, Kim C, Chung WS, Cho JH, Nam D, Kim SH, et al. The hydrolysed products of iridoid glycosides can enhance imatinib mesylate-induced apoptosis in human myeloid leukaemia cells. Phytother Res. 2015; 29(3):434-43. [PMID]
- Jin D, Cao M, Mu X, Yang G, Xue W, Huang Y, et al. Catalpol inhibited the proliferation of T24 human bladder cancer cells by inducing apoptosis through the blockade of Akt-mediated anti-apoptotic signaling. Cell Biochem Biophys. 2015; 71(3):1349-56. [PMID]
- Liu C, Wu F, Liu Y, Meng C. Catalpol suppresses proliferation and facilitates apoptosis of MCF-7 breast cancer cells through upregulating microRNA-146a and downregulating matrix metalloproteinase-16 expression. Mol Med Rep. 2015; 12(5):7609-14. [PMID]
- Zhu P, Wu Y, Yang A, Fu X, Mao M, Liu Z. Catalpol suppressed proliferation, growth and invasion of CT26 colon cancer by inhibiting inflammation and tumor angiogenesis. Biomed Pharmacother. 2017; 95:68-76. [DOI:10.1016/j.biopha.2017.08.049] [PMID]
- Liu L, Gao H, Wang H, Zhang Y, Xu W, Lin S, et al. Catalpol promotes cellular apoptosis in human HCT116 colorectal cancer cells via microRNA 200 and the downregulation of PI3K Akt signaling pathway. Oncol Lett. 2017; 14(3):3741-7. [PMID] [PMCID]
- Wang L, Xue GB. Catalpol suppresses osteosarcoma cell proliferation through blocking epithelial-mesenchymal transition (EMT) and inducing apoptosis. Biochem Biophys Res. Commun. 2018; 495(1):27-34. [DOI:10.1016/j.bbrc.2017.10.054] [PMID]
- Wang ZH, Zhan-Sheng H. Catalpol inhibits migration and induces apoptosis in gastric cancer cells and in athymic nude mice. Biomed Pharmacother. 2018; 103:1708-19. [DOI:10.1016/j.biopha.2018.03.094] [PMID]
- Fei B, Dai W, Zhao S. Efficacy, safety, and cost of therapy of the traditional Chinese medicine, catalpol, in patients following surgical resection for locally advanced colon cancer. Med Sci Monit. 2018; 24:3184-92. [PMID] [PMCID]
- Wang Z, Lu Y, Sheng B, Ding Y, Cheng X. Catalpol inhibits TGF-β1-induced epithelial-mesenchymal transition in human non-small-cell lung cancer cells through the inactivation of Smad2/3 and NF-κB signaling pathways. J Cell Biochem. 2019; 120(2):2251-8. [PMID]
- Zhao L, Wang Y, Liu Q. Catalpol inhibits cell proliferation, invasion and migration through regulating miR-22-3p/MTA3 signalling in hepatocellular carcinoma. Exp Mol Pathol. 2019; 109:51-60. [PMID]
- Qiao PF, Yao L, Zeng ZL. Catalpol mediated microRNA 34a suppresses autophagy and malignancy by regulating SIRT1 in colorectal cancer. Oncol Rep. 2020; 43(4):1053-66. [DOI:10.3892/or.2020.7494] [PMID] [PMCID]
- Wu L, Li H, Chen S, Wu X, Chen X, Wang F. Catalpol inhibits the proliferation, migration and metastasis of HCC cells by regulating miR‑140‑5p expression. Mol Med Rep. 2021; 23(1):29. [PMID]
Type of Study:
Review article |
Subject:
Phyochemistry